- 1Escuela de Ciencias Aplicadas e Ingenierías, Maestría en Ciencias de la Tierra, Universidad EAFIT, Medellín, Colombia
- 2Geological Environment and Tectonics Research Group EAFIT University, Medellín, Colombia
- 3National Museum of Natural History, UMR 208 PALOC, Paris, France
- 4Universidad Nacional de Colombia, Departamento de Geociencias y Medio Ambiente, Medellín, Colombia
- 5Department of Geological Sciences, University of Florida, Gainesville, FL, United States
This study delves into the intricate relationship between Colombia’s status as one of the world’s 17 Megadiverse countries and the socio-environmental challenges the country faces, with focus on the geological diversity of the Sierra Nevada de Santa Marta (SNSM); the world’s highest coastal massif laying in the Caribbean Region of Colombia. Despite its natural splendors and magnificence, SNSM’s ecosystems have been significantly shaped by the enduring Colombian armed conflict (1964- latent today), leading to alarming environmental degradation that affects both geological and biological diversity, and hence the local communities inhabiting the territory. Employing modern geological methodologies such as Remote Sensing and Landscape Metrics, this research explores and quantifies the extent of degradation within the SNSM. The findings, spanning the pivotal years from 2000 to 2020, offer an innovative analysis of the Geo-Bio-Megadiverse ecosystems of the Sierra. This comprehensive examination reveals crucial insights to advancing informed environmental management, while supplying a groundwork for potential geoconservation strategies in this embattled territory; unequivocally acknowledged as a victim of a multifaceted and long-lasting socio-environmental conflict. The definition “territory as a victim” originated by local indigenous communities and is now incorporated in transitional justice systems such as the Jurisdicción Especial para la Paz (JEP, Colombia), condenses the profound violence experienced by the land, one that muted the spiritual guardians inhabiting its waters, trees, plants, soils, and stones. By spotlighting the intricate interplay between social struggles and environmental degradation as a central element of the ecosphere-techonosphere conflict in the Anthropocene, this study heightens awareness of the urgent issues confronting the SNSM, while advocating for targeted conservation initiatives to preserve its distinctive and invaluable natural heritage.
Introduction
A paradigmatic shift in geosciences occurred at the dawn of the 21st Century with the establishment of Earth System Science (ESS) as a necessary starting point for a more holistic understanding of our planet’s functioning. ESS emphasized the intricate interactions between the various spheres, i.e., geo-, hydro-, atmo-, bio-, and even the Technosphere (Steffen et al., 2020; Commoner, 1990). This new approach to study our planet integrates living and non-living entities, thereby contributing to the recognition of geological diversity, or geodiversity, as an essential element in modern geological thinking (Gray, 2005; Gray, 2019). Originating from the Rio Earth Summit in 1992, geodiversity gained formal acknowledgment in 1993, marking a pivotal expansion beyond the traditional focus on biotic diversity. The International Union for Conservation of Nature (IUCN) officially embraced “geodiversity” in 2018, underscoring its importance for environmental management, particularly in protected areas. In recent years, the convergence of geodiversity and biodiversity has become evident, highlighting their joint significance in ecosystem management and conservation (Comer et al., 2015; Pellitero et al., 2016). While biodiversity has traditionally been emphasized for its direct relationship with ecosystem goods and services, geodiversity’s role in critical processes like nutrient cycling and climate regulation has gained recognition. Nutrient cycling, the movement of essential elements through ecosystems, is influenced by geological factors such as soil composition and topography (Noll et al., 2022). Geodiversity also plays a crucial role in climate regulation, affecting carbon storage, albedo, and water cycles (IPCC).1 Consequently, geodiversity indicators are now integral to nature conservation, protected area planning, and broader environmental strategies (Gordon, 2018). Moreover, it is now well accepted that geological process-features of various kinds exert control on shaping biodiversity patterns (Badgley et al., 2017; Antonelli et al., 2018; Sanín et al., 2024).
To understand the intricate relationships within ecosystems that inexorably involve biotic and abiotic elements, the anthropogenic factor—human influence—must be addressed. Since the introduction of the term Anthropocene by Crutzen (2016) in his Nature article Geology of Mankind, or the elaboration on the concept technosphere-ecosphere conflict by Commoner (1990) in the book Making Peace with the Planet, a more thorough understanding of the consequences of human actions on Earth has become vital in effectively addressing ecosystem protection. Despite a lack of comprehensive documentation, these concepts can support innovative programs for ecosystem recovery and resilience studies in the face of natural, anthropogenic, and mixed phenomena, e.g., climate and environmental change (Durán-Izquierdo and Olivero-Verbel, 2021). In Durán-Izquierdo and Olivero-Verbel’s study, the authors delve into a short portion of the Anthropocene period, starting from the early industrial era (∼1850), and with geographic focus on the Sierra Nevada de Santa Marta (SNSM), to examine human impacts, particularly evident in activities such as deforestation (Ruddiman, 2013). Currently available tools, including remote sensing and GIS, have been employed to quantify and assess the effects of these perturbations on ecosystem dynamics (Culbert et al., 2012; Schindler et al., 2015).
Colombia, situated in the Tropical Andes of northwestern South America between the Pacific Ocean and the Caribbean Sea, boasts diverse ecosystems ranging from topical dry to hyper-pluvial, and from highmontane to mangrove, many of which are supported by abundant forests (Spencer et al., 2024). As multiple ecological hotspots converge in this region, including the Caribbean, Mesoamerica, Tropical Andes, and Chocó-Darien-Western Ecuador/Tumbes-Chocó-Magdalena (Mittermeier et al., 1999; Mittermeier et al., 2011), Colombia faces significant challenges in preserving its valuable ecosystems (Myers et al., 2000; Rodríguez et al., 2020). Rapid deforestation, particularly between 1990 and 2005, has led to a substantial loss of forest cover, especially in Amazonia and the Caribbean region (753,893 ha that represents the loss of 4.9% of total forest) (Armenteras et al., 2013). Between 2013 and 2018 alone, deforestation accumulated to ca. 3,482 km2 (González-González et al., 2021). The Sierra Nevada de Santa Marta (SNSM), a coastal mountain range with vast altitudinal gradients of >6,000 masl, harbors a diverse range of litho-structural and geomorphic units, contributing to a rich variety of microclimates, soil assemblages, and fluvial-glacial basins that support numerous ecosystems (Hooghiemstra and Flantua, 2019). However, the SNSM has been significantly impacted by the armed conflict in Colombia, which has been complex and multifaceted involving multiple armed actors (e.g., the army, rebel groups such as FARC-EP and ELN, paramilitary organizations and drug cartels self-defense forces) as well as illicit drug production-trafficking since the 1970s, resulting in the progressive degradation of soils, waters, micro-climates, and landscapes (Álvarez, 2007; Durán-Izquierdo and Olivero-Verbel, 2021; Negret et al., 2019). This degradation has also affected indigenous communities of the SNSM (Wiwa, Kogi, Arhuaco, Arzarios and Kankuamo) as well as larger urban centers such as Santa Marta, Valledupar, and Riohacha, highlighting the interconnectedness of socio-environmental issues across Colombia (Rocca and Zielinski, 2022).
In this paper, we aim to: 1) assess the geodiversity of the SNSM, 2) evaluate the spatiotemporal patterns of deforestation in the SNSM between 2000 and 2020, and 3) explore the interplay between the evolution of geodiversity and biodiversity with social phenomena, particularly the armed conflict and illicit coca cultivation for cocaine production. The study aims to emphasize potential ecosystem effects and proposes tools for integrating Geoconservation into the development of more coherent land planning and environmental strategies and policies for the SNSM.
Study Site: The Sierra Nevada de Santa Marta
The SNSM, located within the jurisdictions of the departments of Magdalena, Cesar, and Guajira, constitutes a significant mountain massif topographically isolated from the rest of the Andean ranges in Colombia (i.e., the Western, Central, and Eastern cordilleras). Emplaced along the northern coast of Colombia and facing the Caribbean Sea, the SNSM resembles a massive, natural pyramid with an almost perfect triangular base measuring approximately 500 km in perimeter, and its northern side running virtually from west to east parallel to the coastline (Figure 1). Some of its peaks reach elevations of ∼6,000 m, including Pico Colón and Pico Bolivar, the country’s highest summits, so that the SNSM is considered the world’s highest isolated coastal mountain (Tschanz et al., 1974; Restrepo-Moreno et al., 2019). This topographic condition is further emphasized if the submarine trench, approximately 4.5 km deep (defining the subduction boundary between the Caribbean and South American Plates) is considered, potentially making “the mountain” over 10 km high. The massive topographic gradients of the SNSM, ranging from sea level to snow-capped summits within less than 50 km, result in a multiplicity of climates and ecosystems. These vary from the hot lowlands of the Caribbean Region and its littoral zones to permanently nival climates at its summits, which are the most northernly ice caps in South America. Additionally, the area encompasses contrasting ecosystems that include estuaries and mangrove patches at its base, and paramos and tundra towards the top. In essence, the SNSM hosts many of the vegetation assemblages observed by Humboldt and/or Caldas during their journeys through tropical America. This astonishing variety of environments represents at least 14 of the life zones of ecology summarized by Holdridge (1967), spanning the full altitudinal range from Premontane to Nival and across climatic-precipitation gradients ranging from very wet to desert, with marked contrasts in mean annual precipitation.
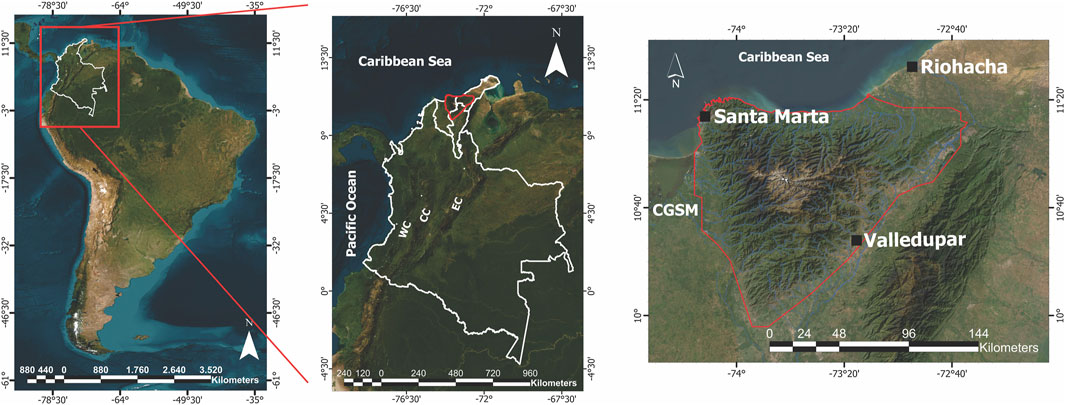
Figure 1. Sierra Nevada de Santa Marta ocation in the South American and Colombian and Caribbean contexts. The three main cordilleras, Western, Central and Eastern are marked as WC, CC, and EC, respectively. The Ciénega Grande de Santa Marta (CGSM) and the cities of Santa Marta, Valledupar, and Riohacha are indicated.
Crust-atmosphere coupling causes the SNSM to work as an efficient trap for atmospheric moisture from the Caribbean Basin, which is transformed into several major fluvial basins. These include the Nabusímake-Fundación, Aracataca, Sevilla, and Frio rivers that flow into the Ciénaga Grande de Santa Marta. Additionally, there are rivers such as the Córdoba, Gaira, Manzanares, Piedras, Guachaca, Buritaca, Don Diego, Palomino, San Miguel, Ancho, Santa Clara, Jerez, Tapias, Camarones, De Moreno, and Ranchería, whose waters directly reach a stretch of the Caribbean Sea coastline from the city of Santa Marta (Magdalena) to Rio Hacha (Guajira). Furthermore, tributaries of the Cesar River in the southeast, including the Ariguaní, Garupal, Dilvio, Los Clavos, Cesarito, Calao, Dunuchuí-Guatapurí, Seco, Badillo, and San Francisco rivers, contribute to the hydrological network of the region (Figure 1). On the other hand, the Sierra serves as the primary water source for three departments (Guajira, Cesar, and Magdalena), producing over 10 billion cubic meters of water annually, which is utilized by ∼1.5 million people (Viloria-de-la-Hoz, 2005), including the native communities (Kogi, Wiwa, Arhuaco, and Kankuamo). These indigenous groups, with their ancestral knowledge, represent the important cultural richness in the SNSM. The cryospheric component of the Sierra’s hydrosphere sustains rapidly retreating ice caps (Poveda and Pineda, 2009). In the last 200 years, 8 glaciers have disappeared in Colombia, with the remaining six experiencing rapid retreat approximal to 70% since 50s.2 Recent geomorphological data from the SNSM indicate a current retreat rate of 0.34 km2/year, suggesting that perpetual snow may vanish in less than 20 years. This loss of glacier mass not only impacts geodiversity but also leads to the disappearance of geosystem services, affecting various ecosystems in the region (Zapata-Herrera et al., submitted). The SNSM is divided into three distinct tectonic provinces (Tschanz et al., 1974). From East to Weast these are: 1) the Sierra Nevada Province, located to the southeast, geologically composed of an ancient granulitic rocks (∼1.3 Ga, Proterozoic), covered by rocks of Paleozoic and Permian (?)–Triassic ages, without evidence of metamorphism, and Jurassic volcanoclastic deposits; this is the only province with this type of deposits. 2) The Sevilla Province, located further north and bound by the Sevilla Lineament, consists of Paleozoic metamorphic rocks intruded by Permian and Late Triassic granites. 3) In the northwest corner lies the youngest one; the Santa Marta Province, comprised of low-grade metamorphic rocks from the Upper Cretaceous intruded by Paleogene granites (Tschanz et al., 1974; Colmenares et al., 2007).
The geodynamical context of the SNSM is related to The Maracaibo Orogenic Float (MOF), that hosts several physiographic units of the Santander Massif, Mérida Range, Perijá Range, SNSM, and the Cesar-Ranchería, Catatumbo, and Maracaibo basins, which collectively form the Maracaibo Block. What is distinctive about the MOF is that it is a portion of the Guiana Shield that extends further to the northwest, covered by extensive Phanerozoic sequences (e.g., Cediel, 2019 and references therein). Due to its position in the NW corner of South America, the SNSM constitutes an area with significant tectonic activity, as it lies at the convergence of several tectonic plates, including the South American, Caribbean, and Nazca plates (Cediel, 2019). This interaction has resulted in various structures such as faults, folds, and thrusts.
Due to its geographic location, geology and topography, the SNSM hosts diverse ecosystems, both marine and terrestrial, ranging from coral reefs to forests, paramos, and glaciers, making it one of the most biodiverse regions on the planet, with a high concentration of endemic species (IDEAM, I., IAVH, S., y IIAP, 2017; Carbonó and Lozano-Contreras, 1997; Fortier et al., 2024). The diverse natural environments of the SNSM, biotic and abiotic, served as the backdrop for the development and flourishing of many significant American cultures before the invasion and genocide perpetrated by Europeans in the late 15th Century. Geodiversity can be attributed as a key factor contributing to the extreme biodiversity and cultural diversity of the SNSM, a reflection that extends to Colombia as a whole (Gadgil, 1987). Presently, at least five different indigenous groups inhabit the SNSM—the Kogis, Aruacos, Kankuamos, Wiwas, and Arzarios (Reichel-Dolmatoff, 1982). Each cultural group has developed distinct modes of interaction with the territory, encompassing spiritual-cosmogonic aspects as well as technical and technological practices related to agriculture, water and soil management, architecture, and more. The presence of coca as an ancestral sacred plant has always played a fundamental role in these cultural practices. Since Dolmatoff’s work, ongoing research continues to uncover evidence of the multiplicity of forms of occupation and sustainable use of the territory. Gutiérrez-Montoya (2022) focused on the Forgotten Cities: Occupation and Architectonic Development, which delve into understanding the occupation of SNSM, and Funari (2023) emphasizes cultural change and environmental awareness.
Since the time of invasion and genocide in the late 1400s, and with a dramatic intensification over the 20th and 21st centuries, the SNSM and its peoples have been subjected to major modifications of their natural and cultural environments, primarily in the form of deforestation and the introduction of European agricultural practices (Manjarrés-García and Manjarrés-Pinzón, 2004; Viloria de la Hoz, 2006; Durán-Izquierdo and Olivero-Verbel, 2021). Wars over the control of land and the illegal drug markets, starting with marijuana in the early 1970s and then coca for cocaine production in the 1980s until today, have continued to impose the horrors of a brutal conflict not only on the people living in that territory but also on the land itself, including plants, animals, rocks/soils, and waters (Britto, 2020). It is this perpetually threatened SNSM territory that we envision as a geological victim, including the humans that inhabit it, as we are, after all, part of the geological evolution of our planet. We do so in the sense suggested by Huneeus and Rueda-Sáiz (2021), where the “territory” constitutes not only the stage on which a specific form of conflict unfolds but also as a victim itself, to the point that it leads to a legal expression of the idea that both human lives and those of other entities, living and “non-living,” are obliterated as a result of war, including “earthy beings” such as rivers, soils, rocks, mountains, and the spiritual world—a set of concepts that are, in fact, intertwined in Colombia’s peace process that seeks to transform the territory “from an object to a legal subject that suffers harm and is in need of repair.” It is this territory-as-a-victim expressed in the SNSM that we seek to help better understand and protect in the context of its ge-diversity, encompassing the geosphere, atmosphere, hydrosphere, and biosphere, which form the supporting structure of all the other diversity expressed in the Technosphere that now places the Mega-Diversity of the SNSM under siege.
Materials and Methods
We employ a combination of geospatial approaches to integrate geological information across different spheres of the Earth System, encompassing the geo- (lithologies, structures, geomorphology, and soils), atmo- (climate and precipitation patterns), hydro- (rivers and glaciers), and biosphere (vegetation cover and biodiversity).
Geodatabase (Gdb) Conformation and Analysis
The creation of a Geodatabase (Gdb) is a fundamental step in understanding the significance of high-value biodiversity data. This approach requires the inclusion of areas within the SNSM that are more comprehensive geologically speaking, and that encompass their evolving dynamics, including their connection with the societal challenges posed by illegal crops and associated warfare. The first phase of this process involves the compilation of both primary and secondary information, sourced from field expeditions, tourism guides, scholarly papers and theses, and other pertinent references.
Geological and geomorphological data primarily originated from 1:100,000 scale maps provided by the Servicio Geológico Colombiano (SGC; Colombia’s geological survey), specifically sheets number 12, 13, 14, 15, 20, 21, 22, 23, 27, 28, 29, 35, and 36. Climate and soil data were extracted from the ecosystem study conducted by the Instituto de Hidrología, Meteorología y Estudios Ambientales de Colombia (IDEAM, I., IAVH, and IIAP, 2017), the base hydrology, composite by the main rivers and swamps, was taken from Instituto Geográfico Agustin Codazzi3 at a 1:100,000 scale.
Geodiversity Quantitative Methods
For the quantitative assessment of geodiversity, we utilized the Geodiversity toolbox proposed by Valentin (2021). We divided the study area into a raster grid of 1.5 km × 1.5 km sections, incorporating information from geological, geomorphological, pedological units, structural geology (represented by faults, folds, and lineaments extracted from DEM-derived hill shades), and hydrology (based on Strahler River classification). These five sub-indices collectively contributed to the calculation of the total geodiversity (number of abiotic elements per unit area). The geodiversity index was computed by map algebra additions of all sub-indices, with equal weights assigned to each variable, following the methodology successfully applied in the Chinchiná River Basin in the Colombian Andes (Arias-Díaz et al., 2023). While the classical approach of using a number density of selected diversity indicators is a common method for assessing geodiversity, it is important to note that different methodologies are available. These alternatives provide relative values for specific diversity indicators, allowing for a more nuanced understanding of geodiversity in different contexts. The ultimate goal of these methods is to generate maps that identify areas with potential geosites, which can be valuable for conservation, education, and tourism purposes (Zakharovskyi et al., 2023; Zakharovskyi et al., 2024).
Morphometric Diversity Map
The morphometric diversity map was based on the index proposed by Argyriou et al. (2016). We focus on six sub-indices: slope gradient (Sg), dissection index (Di), stream frequency (Fu), drainage density (Dd), and Topographic Wetness Index (TWI). This analysis was implemented on a grid of 404.496 km2 and aimed to accentuate geomorphological, hydrological, and morphotectonic settings in this tectonically active region. Morphotectonic information, crucial for this analysis, was derived from the amplitude relief index (Ar), describing fluvial erosion and vertical displacements. Geomorphological processes were assessed by Sg and Di, while hydrological indicators (Fu, Dd, and TWI) provided insight into surface runoff potential, geomorphic activity, landscape dissection, and soil moisture. Normalization, summarization, and the application of the Natural Breaks function were carried out to highlight areas with anomalous morphometric indices, indicative of susceptibility to degradation related to land cover changes.
Land Cover Changes Estimations
The geodatabase of land cover changes utilized raster data from Hansen Global Forest Change v1.8 (Hansen et al., 2013), processed using the Python API in Google Earth Engine (GEE) to analyze deforestation processes from 2000 to 2020. This timeframe is significant as it spans the transition from the peak of the armed conflict to the initiation and implementation of the Peace Agreements in 2016. The raster data, classified into 21 classes, underwent further analysis using the ArcGIS Pro calculator to quantify forest area loss in pixels (30.92 m × 30.92 m), which was then normalized to 10,000. To identify critically deforested regions, a basin-to-basin comparison was conducted, aiming to identify the most vulnerable basins and assess their level of criticality in terms of deforestation. Land cover is crucial not only in biological terms as it directly implies loss of habitat and hence of biodiversity (Haines-Young, 2009), but also a key influence on the hydrological-fluvial and geomorphic behavior (erosion, natural slope stability) of mountainous regions (Alvarado-Villalon et al., 2003).
Results
Gdb Analysis
Numerous studies conducted in the SNSM, and surrounding areas (Figure 2) underscore the importance of this unique lithostratigraphic province. The geological records in the SNSM span from the Precambrian to the present day, capturing orogenic processes associated with the assembly of supercontinents Rodinia, Gondwana, and Pangea. This area has been a focal point for some of the country’s earliest geodiversity studies, with many locations of geological interest identified. Among the documented studies compiled in the SNSM Geodatabase, 71% have been published in international journals (e.g., Journal of South American Earth Sciences, International Geology Review, Geologica Acta, GSA Bulletin, Sedimentary Geology). The remaining 29% are tied to thesis projects from universities that include EAFIT (Escuela de Administración, Finanzas e Instituto Tecnológico or by its translation School of Administration, Finance and Technological Institute) University (primarily focusing on the geodiversity zoning), Universidad Nacional de Colombia (Colombia) and Université Grenoble Alpes in France (Figure 3). These studies emphasize the geological significance of the SNSM, capturing a wide diversity of geological processes at various temporal-spatial scales. Work accumulated over several decades, but particularly over the last 20 years, has resulted in a comprehensive overview of the geological history within the national territory. Most geological-related data for the SNSM (geo-, atmo-, hydro- and bio-spheric) is available at a 1:100,000 scale or was collected at the same scale, forming a foundational dataset for calculating and zoning geodiversity. Collected data were then used to create a Geodatabase of Geological Interest Points (GIPs). The choice of these 128 points considered criteria such as scientific, geomorphological, ecological, or cultural relevance. These points serve as tangible, real-world examples of the evolution of this geological complex and its tectonic framework. They also act as proxies for finding different “bio-geo-cultural hotspots,” contributing to the development of effective management plans, including water and ecosystem regulation, and the integration of eco-tourism (turismo ecológico/turismo de naturaleza). Examples of such key locations include: Pico Bolivar (the highest summit in the SNSMa tca. 5780 masl), Ciudad Perdida (ruins of an ancient Kogi settlement), indigenous reservations (e.g., Kankuamo, El Zahino-Guayabito-Muriaytuy, Potrerito), the glacier deposits, the beaches along the Tayrona National Park, the main rivers descending from the SNSM, among others.
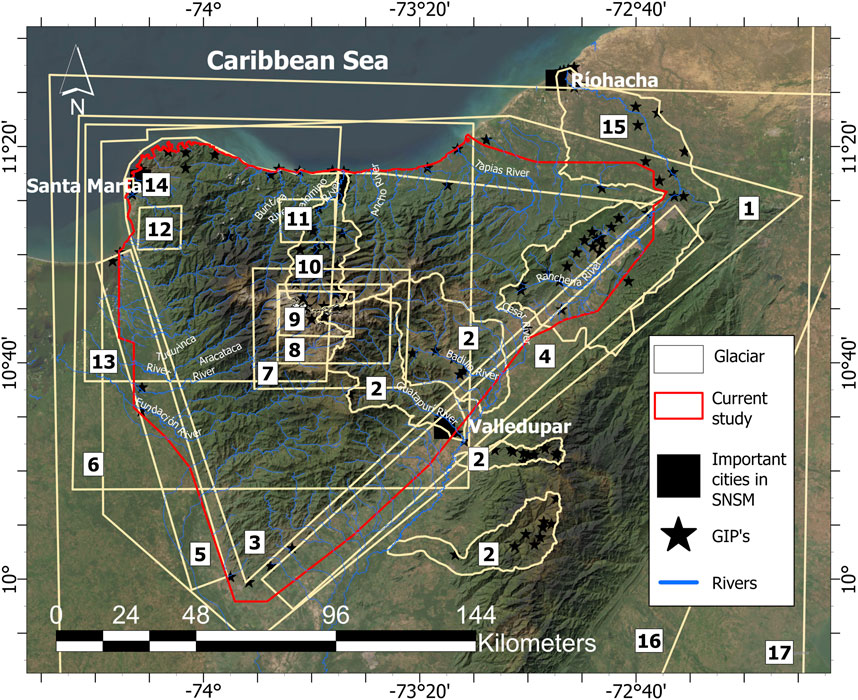
Figure 2. Map of studies undertaken at the SNSM: 1. Tschanz et al. (1974); 2. Valencia-Jaramillo and Castañeda-Molina (2020); 3. Duque-Trujillo et al. (2019); 4. Bayona et al. (2006); 5. Idárraga-García and Romero (2010); 6. Piraquive-Bermúdez (2016); 7. Pizarro-Bello (2019); 8. López-Moreno et al. (2020); 9. Poveda and Pineda (2009); 10. Gutiérrez-Martínez (2021); 11. Cardona et al. (2008b); 12. Cardona et al. (2008a); 13. Piraquive et al. (2022); 14. Bustamante et al. (2009); 15. Pérez and Naranjo (2021); 16. Wokittel (1957); 17. Quiroga et al. (2024). The GIPs Inventory is marked with black stars. The red polygon marks the current study.
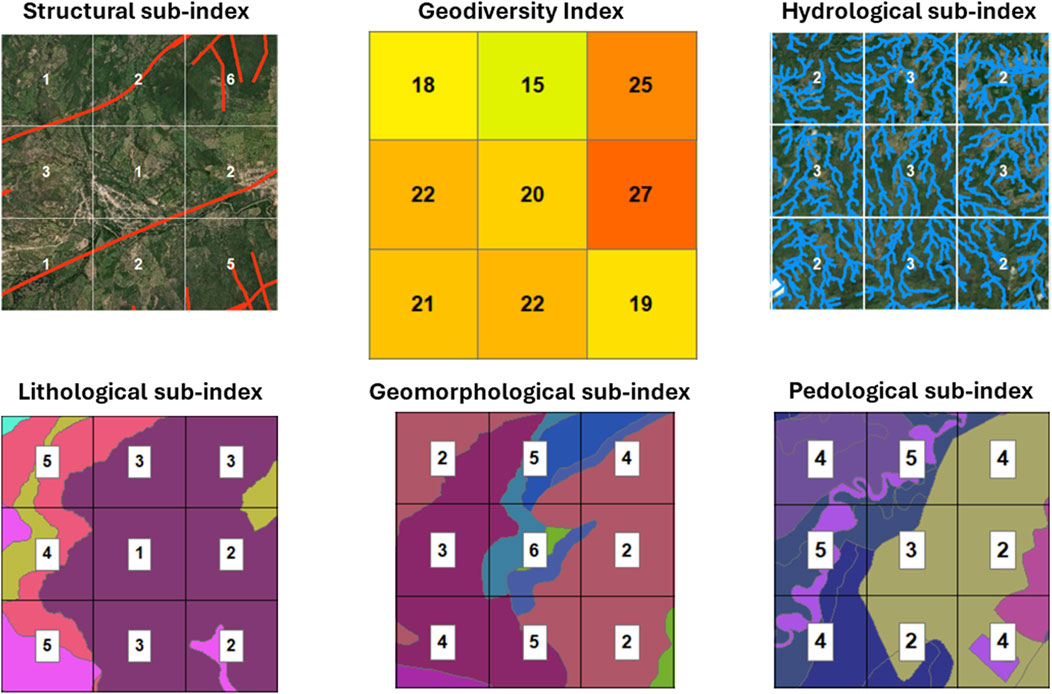
Figure 3. Geodiversity estimation framework. Adapted from Valentin (2021).
The SNSM boasts exceptional geodiversity. This complex geological entity features Precambrian crystalline basement rocks overlain by Paleozoic and Mesozoic sedimentary formations, contributing to the region’s unique mineral resources and scenic landscapes. Home to four indigenous communities—the Arhuaco, Wiwa, Kogi, and Kankuamo—the Sierra Nevada serves as a cultural and spiritual heartland. Recognizing their traditional land stewardship, several indigenous reservations exist within the mountain range. Additionally, the Sierra Nevada de Santa Marta National Natural Park, established in 1964, safeguards a significant portion of the region’s ecosystems, aiming to conserve its biodiversity and the cultural heritage of indigenous communities. Understanding the geodiversity of the Sierra Nevada de Santa Marta is crucial for effective conservation strategies, highlighting the intricate interplay of geology, cultural heritage, protected areas, landforms, soils, and life zones that make it a truly unique and irreplaceable environment.
Geodiversity Estimation
In the SNSM, several conservation figures with different aims coexist (Henao and Molina, 2010), such as the Biosphere Reserve Area, the 19 indigenous reserves, and the two National Natural Parks (Sierra Nevada de Santa Marta and Tayrona), which also have development zones that include 152 mining operations concentrated along the edges of the Sierra. The indigenous reserves and National Natural Parks are mainly found in the higher parts and encompass littoral areas towards the NW corner of the SNSM (e.g., Tayrona). Despite these administrative efforts, the native communities at the SNSM are at conflict with mining operations, mainly due to socio-environmental degradation caused by the latter (Figure 4).
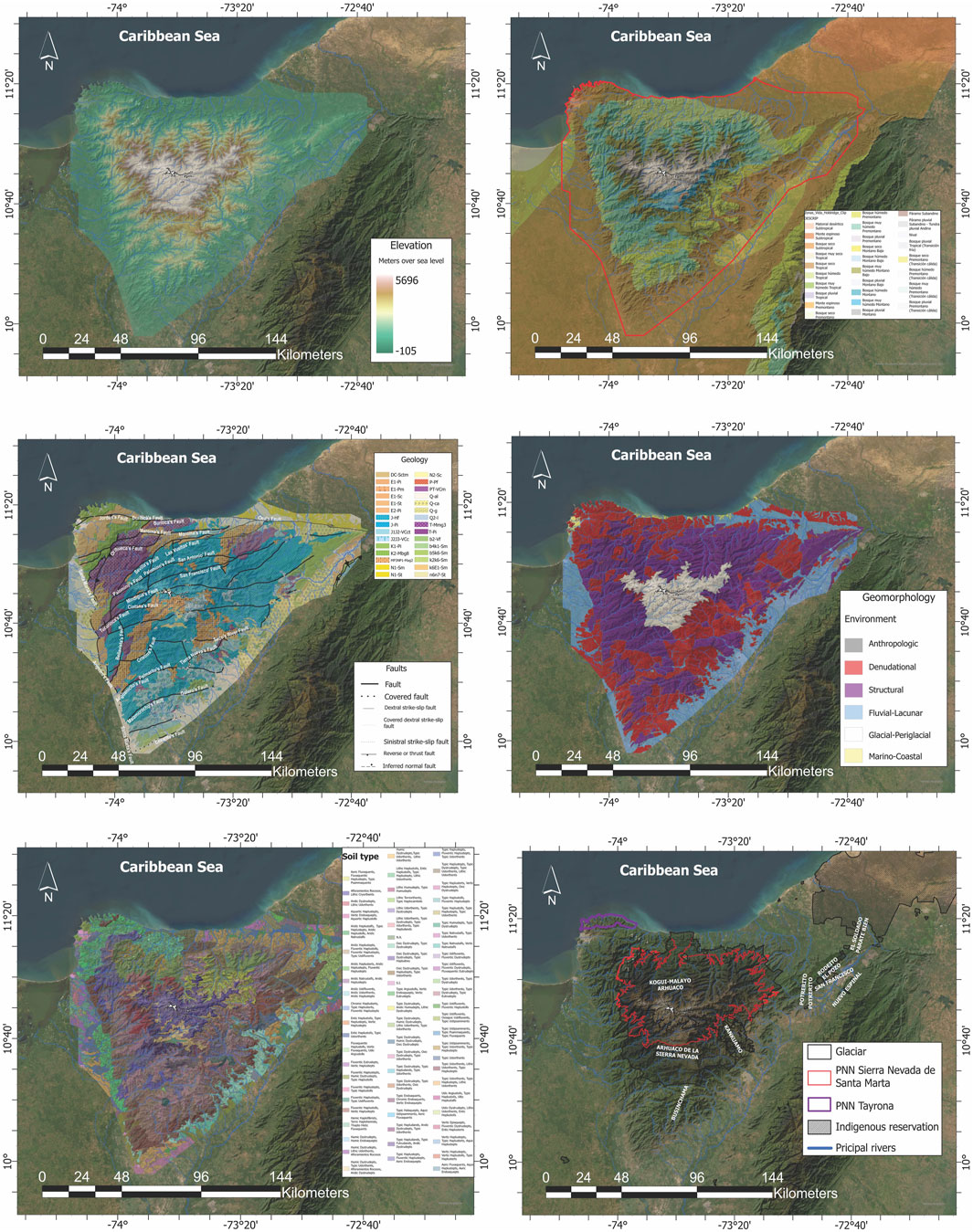
Figure 4. Elements of the SNSM geo-diversity. Altitude, lithologies, soils, life zones, geomorphology and indigenous groups.
In total, 33,188 abiotic elements (polygons and lines) were found in the SNSM covering ca. 1,732,435.35 Ha. The distribution is listed in Table 1. In the Caribbean portion of the SNSM, a pronounced rainfall gradient relates to the marked topographic barrier imposed by this massive mountain (orographic precipitation). Rainfall varies from around 500 mm/year near coastal zones to 3000 mm/year along the high mountains (Manjarrés-García and Manjarrés-Pinzón, 2004). The SNSM region is characterized by two distinct climatic periods, a rainy season (September-November and May-June) and a dry season (January-April and July-August) (Serna et al., 2015). Warm climates (arid and semi-arid, humid, and semi-humid) prevail in the lowland and coastal areas (56.12%), with the most extensive being the warm semi-arid climate (25.95%).
The survival of key ecosystems, such as the páramos, which are endemic ecosystems of the Andes (Cuatrecasas, 1968; Balslev and Luteyn, 1992; Pabón and Hurtado, 2002; Hofstede et al., 2003) are threatened by the reduction and eventual disappearing of glaciers of SNSM. Other key ecosystems such as the dry tropical forest (in the process of desertization) and tropical moist mountain forest (transformed into savanah) are also under pressure (Cavelier et al., 1998; Le Houérou, 2002; Mayr et al., 2013). These situations have significant consequences for the stability of the region’s ecosystem assemblages, which have been identified as part of one of the most critical biodiversity hotspots (Myers et al., 2000).
In terms of geomorphological, lithological, and structural diversity, the predominant environments are structural, influenced by the strong tectonic control exerted over the Sierra by the complex geodynamic milieu where it evolved (Cediel, 2019). This is clear through crustal scale structures such as the NW-SE Bucaramanga-Santa Marta Fault to the west, the NE-SW Cesar-Ranchería Fault to the east, and the Oca Fault to the north with an EW direction. Smaller lineaments and faults also follow these preferred orientations, although the NE-SW constitutes the main litho-structural grain of the Sierra (Figure 2), with structure often separating blocks of different lithologies. Denudational environments are also present, controlled by the topography, lithology, soils, vegetation cover, and diverse climatic conditions across the SNSM.
The morphoclimatic diversity of the SNSM significantly impacts its geomorphological features. This diversity ranges from arid lowlands to humid páramos and nival-glacial zones, creating a complex interplay of climatic and geological factors. Orographic precipitation patterns contribute to varied rainfall distribution, shaping the landscape and influencing erosion and soil formation-destruction processes. These diverse climatic conditions, combined with the tectonic and structural influences, result in a unique environment where different ecosystems, such as páramos, thrive amidst the dynamic interactions between climate and geology. Understanding these morphoclimatic factors is crucial for developing effective conservation strategies in this biodiverse and geologically complex region.
Predominant outcropping rocks exhibit compositions ranging from acidic to intermediate with Mesozoic to Triassic-Jurassic ages. To the west, there are granodioritic, quartz-monzonitic, and alkali granite bodies of Triassic age intruding metamorphic rocks of Precambrian age (Stenian-Tonian), corresponding to the Mangos Granulite unit in the central zone and the Buriticá Neiss in the north. Pyroclastic rocks were deposited on these formations. Sedimentary rocks of Permian-Triassic age are also present in the central zone of the Sierra, forming small enclaves known as the Corual Formation. Additionally, sedimentary rocks of Cenozoic age are concentrated in the northeastern, western, and to a lesser extent, in the southern parts of the massif, e.g., Guatapurí and Monguí formations and the Cerrejón Formation found to the east of the Sierra. In the northern portion of the Sierra, Cenozoic igneous bodies such as the Santa Marta Batholith, and the Buriticá and Toribío plutons, intrude metamorphic bodies of Upper Cretaceous age like the Taganga Phyllite and the Gaira and San Lorenzo schists. Most unconsolidated deposits are of Quaternary age and are found along the edges of the SNSM and internally, associated with fluvial basins, along with other Holocene deposits present along the western border (e.g., Tschanz et al., 1974; Cediel et al., 2003; Bermudez, 2016 all references there in).
According to soil studies by (IDEAM), soil types at the SNSM include five of the main orders (Ultisols, Vertisols, Mollisols, Inceptisols, Entisols). More specific types such as Typic Udorthents, Typic Dystrudepts, and Typic Eutrudepts, have a relatively wide distribution along the edge of the Sierra (covering 7.4%), with an exception in a northern stretch. Other soil types like Typic Ustorthents, Lithic Ustorthents, and Typic Haplustepts have a similar distribution, occupying a slightly larger area (8.8%). Soil types including Typic Dystrudepts, Humic Dystrudepts, Lithic Udorthents, and Typic Udorthents are concentrated mainly in the northern and western parts of the Sierra (4.3%). In the central zone (mostly the current and inherited glacial and periglacial areas), rocky outcrops are present instead of soil. In the southwestern zone, soil types such as Typic Dystrudepts, Oxic Dystrudepts, and Typic Udorthents predominate (9.8%) (IDEAM, I., IAVH, S., y IIAP, 2017). In the coastal, lowland, and mid-mountain units, the ecosystems that predominate are those transformed by anthropogenic activity, whether partially or entirely, primarily for agriculture or livestock, which have radically transformed the soils through compaction and erosion.
Abiotic Elements
The number of abiotic elements per unit area in the SNSM zone ranges from 1 to 24. The highest values (between 18 and 24) are concentrated at the center of SNSM, dominated by the main rivers and many crustal structures (predominantly lineaments), while the areas with less abiotic elements are located towards the borders of the SNSM due to the absence of structures and rivers with high order, however in these zones there are presence of multiple Geological Interests Points (GIPs) (Figure 5A). The maps containing the number of abiotic elements, were transformed to a raster format in GIS and underwent a reclassification process using the Natural Breaks method with the Reclassify function. This resulted in a reclassified map with values ranging from 1 to 5, i.e., exceptionally low, low, medium, high, and extremely high (Figure 5B). With this approach geodiversity hot spots appear more clearly in their spatial distribution. The reclassified quantitative method revealed that almost 14.68% of the SNSM belongs to the medium geodiversity zone, followed by 48.75% with High and Exceedingly high. Within the Sierra Nevada National Natural Park, we can recognize the highest values of geodiversity associated with the glacial morphology and the remaining glaciers.
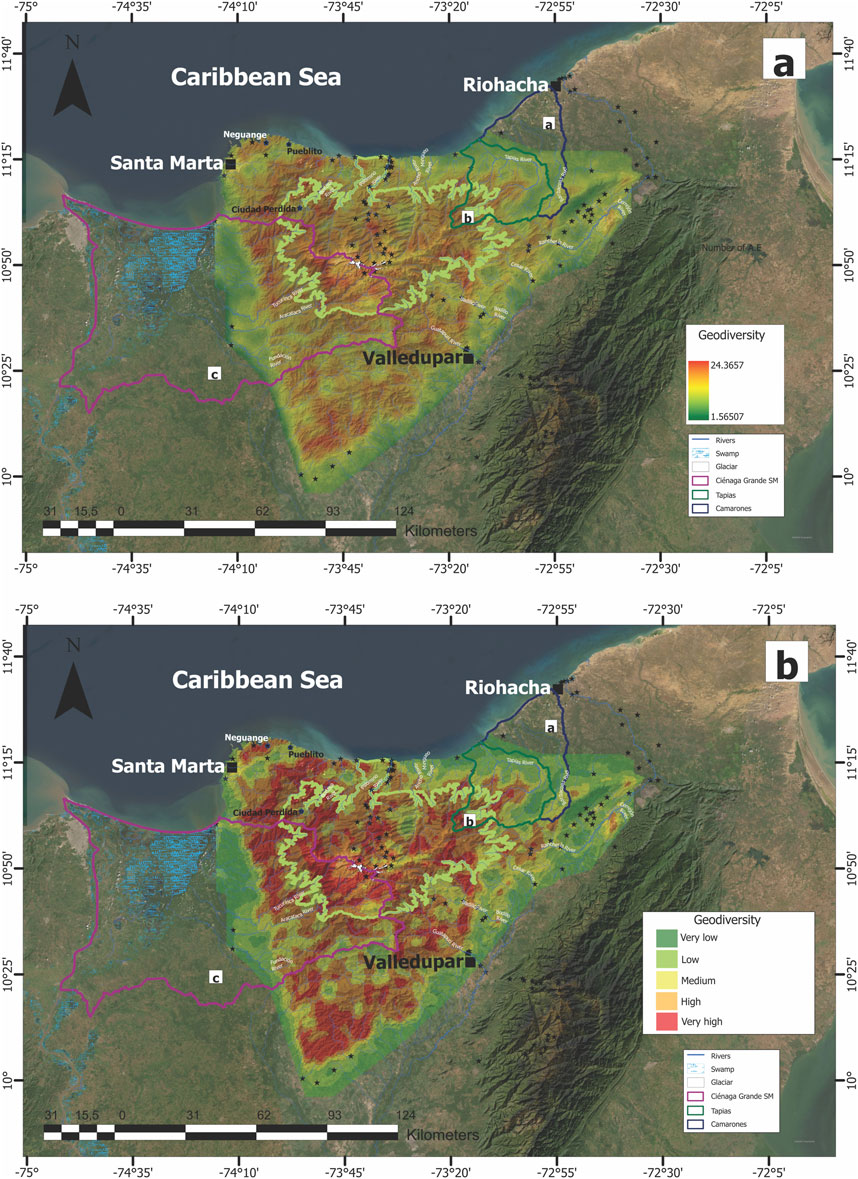
Figure 5. Distribution of quantitative and reclassified geodiversity classes (Abiotic Elements). The black stars represent the LIG’s, the black squares the principal cities and blue circles are the principal populations.
Morphometric Diversity Map
The morphometric diversity map (Figure 6) shows that the H igh and Exceedingly H igh values make up 12.33%. In these zones, there is a confluence of multiple geological processes such as fluvial erosion-dissection, landsliding uplifted or subsiding blocks, and the vertical escarpment of the hill slope. In summary, these areas reflect the evolutionary dynamics of the landscape, aligning with the geologically nature of the SNSM. These characteristics make these areas susceptible to terrain and land cover transformations (Yu et al., 2007). Most of the Geological Interest Points (GIPs) align with these areas showing high Morphometric Index diversity, reasserting the significance of these points in describing the evolution of the SNSM and its unique landscapes and ecological niches.
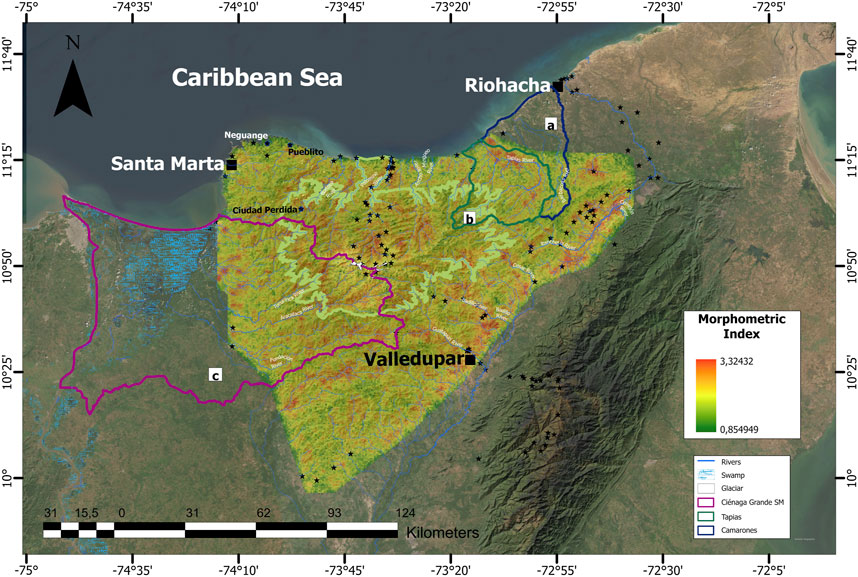
Figure 6. Morphometric Diversity Map. The black stars represent the LIG’s, black squares mark the location of major cities, and blue circles indicate smaller settlements.
Land Uses Changes
Vegetation is a key component in the functioning of earth’s systems, as it participates in important fluxes of mass and energy between the atmosphere, the hydrosphere, the geosphere and the biosphere (DeFries, 2008). From the geomorphic perspective, vegetation exerts strong controls on surface processes such as weathering and erosion, slope development, therefore impacting the status of geodiversity beyond the geosphere, as increased sediment loads in the fluvial network affect riverine flora and fauna and can be transferred to wetlands and estuaries, with deleterious effects on their proper functioning (Wynn et al., 2004; Marston, 2010). Vegetation is also one of the components of the earth’s system more vulnerable to radical modifications from the Technosphere (Houghton, 1994; Fischlin et al., 2007). Loss of vegetation via deforestation is one of the major causes of landscape modifications and environmental degradation, often causing major increases in erosion (Tsegaye, 2019) and the development of badlands and desertization (Ballesteros-Cánovas et al., 2017) and slope destabilization (van Beek et al., 2008). Increased erosion due to removal of the vegetation cover is worse in regions of steep slopes and with marked hydroclimatic gradients, two conditions that are prevalent at the SNSM. The SNSM has been the focus of intense transformation of its natural vegetation (Cavelier et al., 1998; Durán-Izquierdo and Olivero-Verbel, 2021). Most of the original forest covers of the SNSM have undergone some form of modification (Figure 7 shows the deforestation of the main fluvial basins in Sierra Nevada between 2001 and 2020). Concentrating along the main streams and near the coastal area, the Camarones basin (Figure 7A) exhibits a loss of natural cover of ca. 7.19% of the total area, mainly due to agriculture and livestock activities. The principal peaks in deforestation occurred in 2003 with ca. 8.23% of deforestation, and between 2016 and 2018 (26.4% of total cover loss during those 3 years). The Tapias Basin, with the highest deforestation at the northern and eastern flanks, accounts for 11.04% of the total area (Figure 7B), largely impacted by anthropogenic activities such as banana plantations and cattle, especially during the interval 2016–2020. Deforestation rates dramatically increased since 2014, with 58.38% occurring in just 7 years, comparable to earlier peaks such as in 2003 (9.32% of total cover loss). The basin most affected is the Ciénaga Grande de Santa Marta (Figure 7C), particularly in its western zone, due to the expansion of banana plantations. This agricultural activity replaced the natural vegetation covers essential for the mangrove ecosystem proper functioning, while also leading to significant increase in use of agrochemicals now accumulated in both soil and water and in a variety of organisms. Many of these changes occurred during 2016–2020, with the percentage of total deforested areas amounting to 8.21% (see the summary in Table 2).
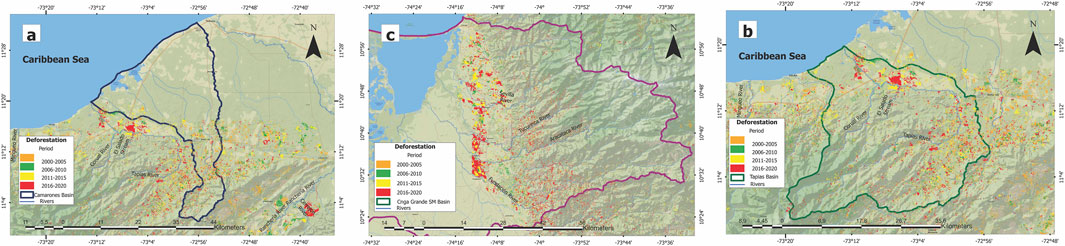
Figure 7. Deforestation in the most affected basins in Sierra Nevada de Santa Marta: Camarones basin (A), Tapias’s basin (B) and Ciénaga Grande de Santa Marta (C).
From the above data, SNSM emerges as a laboratory for studying socio-environmental processes, particularly those related to internal war and conflicts arising from competing land use vocations (agriculture, mining, tourism), a milieu conducive to ecosystem degradation. The loss of natural tree cover, primarily driven by the introduction of illicit crops, and more recently by other land uses like agriculture and cattle grazing. This accelerated deforestation poses a threat to the biomes and biota composition. The deforestation trend shows peaks between 2016 and 2018, with 43.3% occurring during this period. Although the rate started declining in later years, occasional high peaks are still observed.
The SNSM serves as an ideal site for investigating socio-environmental dynamics, particularly those arising from prolonged armed conflict and competition over land use, leading to ecosystem degradation (Jiménez et al., 2015). Deforestation rates have shown an upward trend, with peaks observed between 2016 and 2018, during which 43.3% of the total deforestation occurred. The year 2017 witnessed the highest number of hectares deforested. Although the rate has started to decline in recent years, occasionally high peaks remain evident. The most visible impact of these processes is the significant loss of natural tree cover, with massive deforestation observed since the 1980s, largely driven by expansion of illicit crops (Uribe, 2005). Approximately 90% of the total forest area has been converted to other land uses, such as agriculture, livestock farming, and related activities (Durán-Izquierdo and Olivero-Verbel, 2021). These figures are consistent with those of other authors that indicate that SNSM is one of the most threatened tropical forests due to rapid deforestation, leading to biome degradation and loss of biodiversity (Carbonó and Lozano-Contreras, 1997).
The high rate of deforestation in the SNSM leads to several environmental issues. Firstly, it endangers a significant portion of the forest ecosystems of the Caribbean Ecological Hotspot, a region already at great risk of disappearing (Maunder et al., 2008). Secondly, it contributes to the decline or eventual loss of biodiversity hotspots, as reported by Myers et al. (2000), who noted that 50% of vascular plants and 42% of vertebrates in the area have experienced such declines. Additionally, the integrity and health of communities, soil, and eco-geosystems are compromised by anti-drug policies that employ a war approach supported on herbicide spraying (Peterson, 2002). These policies have failed to effectively reduce drug production and have caused substantial damage to Colombia’s ecology and its most vulnerable citizens (Tokatlian, 2003).
The correlation between geodiversity and deforestation reveals a direct connection between the two factors. We identified three main affected basins, all of which encompass geodiversity hotspots. Specifically, areas with high and extremely high geodiversity are concentrated within the Ciénaga Grande de Santa Marta basin, notably along the Sevilla River and between the Tucurinca and Aracata rivers. Within the Tapias River basin, we observed two additional hotspots, albeit with smaller areas: one over the Tapias River and another between the Corual River and El Salado creek. This scenario underscores a serious concern, as it highlights the vulnerability of geodiversity in the face of deforestation that has occurred over the past two decades. The focal point of this degradation is near these stream systems.
Geodiversity Loss Linked to Deforestation
Gray (2004, 2013) defines geodiversity as encompassing crucial interactions between abiotic elements, emphasizing their significance as the substrates of life and a key component of the interactions of the “living” with the environment. These biotic-abiotic interactions act as drivers of biodiversity, as geodiversity provides access to resources both temporally and spatially. Improved accessibility offers species better opportunities, thereby reducing competition and buffering populations against stochastic events. This implies that a diverse array of abiotic features fosters and sustains greater biodiversity (Parks and Mulligan, 2010).
Threats to geodiversity at the SNSM can be identified through the analysis of land cover changes and land use patterns. In this specific context, one of the primary land cover changes is linked to deforestation, particularly associated with expansion of illegal crops such as marijuana and coca since the 1970s and 1980s, respectively. More recently, the advance of agricultural frontiers, notably banana and cocoa crops, has also contributed to deforestation (Cavelier et al., 1998; Britto, 2020). Both sources of deforestation, illegal and legal, serve as major drivers of environmental transformation, posing threats to geodiversity. These changes can impact abiotic elements such as landforms, soils and water, as well as the geo-services they provide. Additionally, local biota may also be disturbed as a consequence of these transformations (Schwanz et al., 2023). Moreover, geodiversity, as an integral component of the Earth System, can be impacted by the reduction of biodiversity. For instance, the erosion rates and the intensity of the hydrological cycle could be influenced by the loss of natural covers (Maslin and Lewis, 2015). Comprehending geodiversity in mountain systems like the SNSM is crucial for raising awareness of their vulnerability and for understanding biota adaptation (Gordon, 2018). Additionally, mountains serve as significant biodiversity hotspots (Antonelli et al., 2018).
The very high geodiversity within the deforested areas is strongly related to the periphery of the three most affected basins: Camarones, Tapias, and Ciénaga Grande de Santa Marta basins to the north and west. In contrast, in the central zone towards the core of the SNSM, there seems to be no direct relationship between geodiversity and deforestation, partly because forest cover is sparser as the páramo and nival environments become more prevalent (Figure 8A). Nevertheless, the Morphometric Diversity Map, in conjunction with the most heavily deforested areas (Figure 8B), reveals that the most vulnerable regions associated with fluvial-tectonic processes are undergoing significant land use transformations. These alterations have the potential to impact the evolution of hillslopes, potentially increasing runoff and concentrated erosion, which could lead to destabilization of soil-rock massifs and further enhance soil erosion/slope instability, establishing a vicious cycle that may lead to the formation of badlands. Deforestation, recognized as a major driver of erosion rates, may further exacerbate these effects. Such changes can have cascading impacts not only on geomorphic dynamics but also on biodiversity, including fish and herpetofaunal assemblages and bird species richness and abundance (e.g., Werner et al., 2018; Martensen et al., 2012; Suazo-Ortuño et al., 2008).
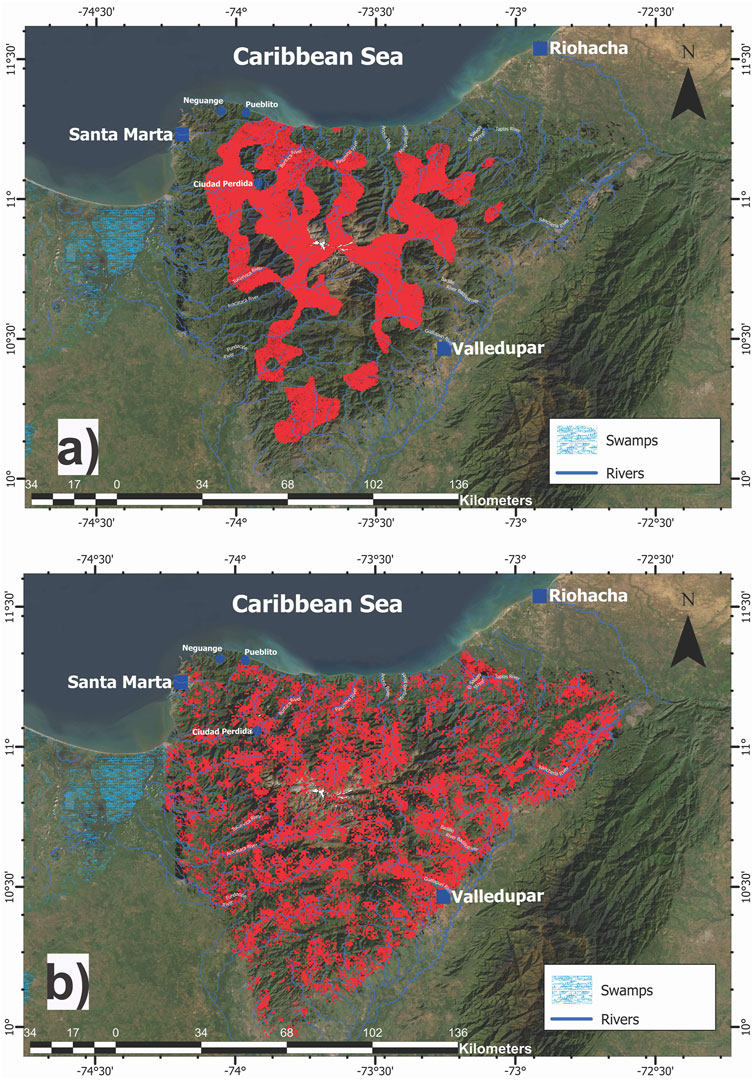
Figure 8. Relationship between geodiversity, morphometric diversity, and deforestation: (A) high geodiversity areas, (B) high Morphometric diversity Index and deforestation in SNSM (the black polygons represent the deforested areas). Red zones are areas with very high diversity. Blue squares show the location of main cities and blue circles mark the location of small towns.
Discussion
Territory as a Victim
The assessment of natural heritage in the SNSM is crucial. This mountain range stores a significant portion of the orogenic history and processes responsible for shaping the Andes in its most northerly expression. Due to its unique position and configuration, the SNSM hosts all ecological zones with their corresponding biological representations, leading UNESCO to declare it a Biosphere Reserve in 1979.4 However, social conflicts in the SNSM, driven by land use changes associated with sociopolitical-socioeconomic instability, contribute to loss of geodiversity as ecosystem dynamics are disrupted and traditional land management practices are compromised. Several examples illustrate this:
Increased Social Conflict: Social conflicts often correlate with changes in land use, including deforestation, agricultural expansion, and infrastructure development (Arias et al., 2014). These transformations can directly affect the geodiversity of the region by altering natural processes that occur in landscapes and ecosystems (Corrales et al., 2019).
Disruption of Traditional Practices: Social conflict may result in the displacement or disruption of indigenous and local communities that have developed traditional and sustainable land management practices that support geodiversity, such as agroforestry and rotational agriculture, practices that may end up being replaced by more intensive and less ecologically sensitive activities (Antrop, 2006).
Loss of Biodiversity Hotspots: The SNSM is renowned for its high biodiversity and unique ecosystems. Social conflicts leading to habitat destruction, fragmentation, or degradation may be conducive to loss of biodiversity in key hotspots and specialized niches, contributing to a decline in overall geodiversity (Jha and Bawa, 2006; Faria et al., 2023).
Altered Water Management: Disputes over water access and usage may trigger modifications in river courses, affecting aquatic ecosystems and associated geodiversity (Zhang and Wei, 2021). Increased erosion and sediment production affect the dynamics of the fluvial system, with consequences throughout the river network and particularly affecting wetland ecosystems in the lowlands and the littoral zone (e.g., riparian forests, mangrove stands, proximal coral reefs).
Soil Degradation: Intensified land use, especially in the context of social conflicts, often causes soil degradation through erosion and nutrient depletion. Changes in soil quality can further affect the distribution of plant species making the lasndacpe more pronoe to evolt into badlands wher hig rates of erosion and deterioratoni of soul fertility further enhance the risks for savanization and or desertizatioun, two situations that in turn negatively influence the overall geodiversity of the area (Lal, 1996; Sarmiento, 2000; De la Paix et al., 2013).
Fragmentation of Ecological Corridors: Fragmentation can disrupt natural processes, reducing genetic exchange among populations and potentially leading to the decline of certain species of flora and fauna (Kupfer and Franklin, 2009). New biotic assamblages may also mean reacomodatinos of the non-biotic elements of the landscape (Oyuela-Caycedo, 2008).
Erosion of Indigenous/Local Knowledge: Indigenous knowledge often includes sustainable land use practices that have helped maintain geodiversity. The loss of such wisdom may further worsen the negative impact on the region’s geodiversity (Oviedo et al., 2007; Goyes et al., 2021).
Vegetation plays a pivotal role in controlling several geologic processes that directly affect the abiotic components of the environment (soils, slopes, water courses, lakes and oceans). Removal of the vegetation cover via deforestation is a major contributor in shifting geomorphic dynamics in mountainous, topical settings (Nyssen et al., 2009). Overall, the years with the highest deforestation in the SNSM were 2017 (13,361.99 ha) and 2003 (10,222.94 ha), each representing an extension of ∼1.4% of the total area of the Sierra. Illicit crops are a significant factor contributing to deforestation in the SNSM, with historical events such as the “Bonanza Marimbera” and “Bonanza Coquera” exacerbating the degradation of the Sierra’s natural system (Calderon-Luna and Torres-Guisao, 2017; Britto, 2020; Gutiérrez-Villegas, 2024). Since the 1990s, violent confrontations and harassment of civilian populations by armed groups have further intensified, altering the landscape, and contributing to the loss of geo- and biodiversity (Díaz-Escobar and Sánchez-Torres, 2004).
The SNSM became a focal point of hostilities between different Colombian armed groups, such as guerrillas and paramilitary organizations like The United Self-Defenses of Colombia (AUC), and drug traffickers vying for control of this strategic zone. The Revolutionary Armed Forces of Colombia—People’s Army (FARC–EP) used the SNSM as a rearguard for the Caribbean Block and as a hiding place for hostages. The AUC repeatedly attacked the civilian population to gain control of the territory, while drug traffickers viewed the SNSM as an ideal territory for producing marijuana and coca, using it also as a corridor and port for smuggling narcotics to the Caribbean and Central and North America (Britto, 2020; Vicepresidencia de la República, 2002).
The data presented highlights the urgent need for stronger enforcement of environmental regulations and governance in the SNSM. The weak presence of governmental entities in the region has further compounded this problem, allowing illegal armed groups to operate with impunity and exert violent influences in the territory. Efforts to strengthen governance structures, increase law enforcement capabilities, and enhance coordination among relevant authorities are crucial to combating these illegal activities and preserving the bio-geo diversity and ecosystem services of the SNSM. Additionally, it is essential to engage local communities in conservation initiatives and promote sustainable land use practices that support ecosystem health and resilience. By empowering communities to participate in decision-making processes and providing alternative livelihood options, we can foster a sense of ownership and stewardship over the region’s natural resources.
Addressing the underlying drivers of deforestation and environmental degradation in the SNSM requires a multi-faceted approach that integrates environmental protection, social equity, and economic development. Collaborative efforts involving government agencies, civil society organizations, indigenous communities, and other stakeholders are essential to safeguarding the Sierra Nevada de Santa Marta for future generations.
The assessment of natural heritage in the Sierra Nevada de Santa Marta (SNSM) highlights not only the region’s biodiversity but also the significant geological features that make it unique. Figure 9 demonstrates the extent of deforestation, particularly due to illicit crop cultivation and land use changes. These images illustrate the impact of human activities on the region’s delicate geodiversity, emphasizing the urgent need for sustainable land management practices. As traditional methods are disrupted and ecosystems fragmented, the SNSM’s rich natural and geological heritage faces increasing threats, making it crucial to address environmental degradation and preserve its unique ecological balance.
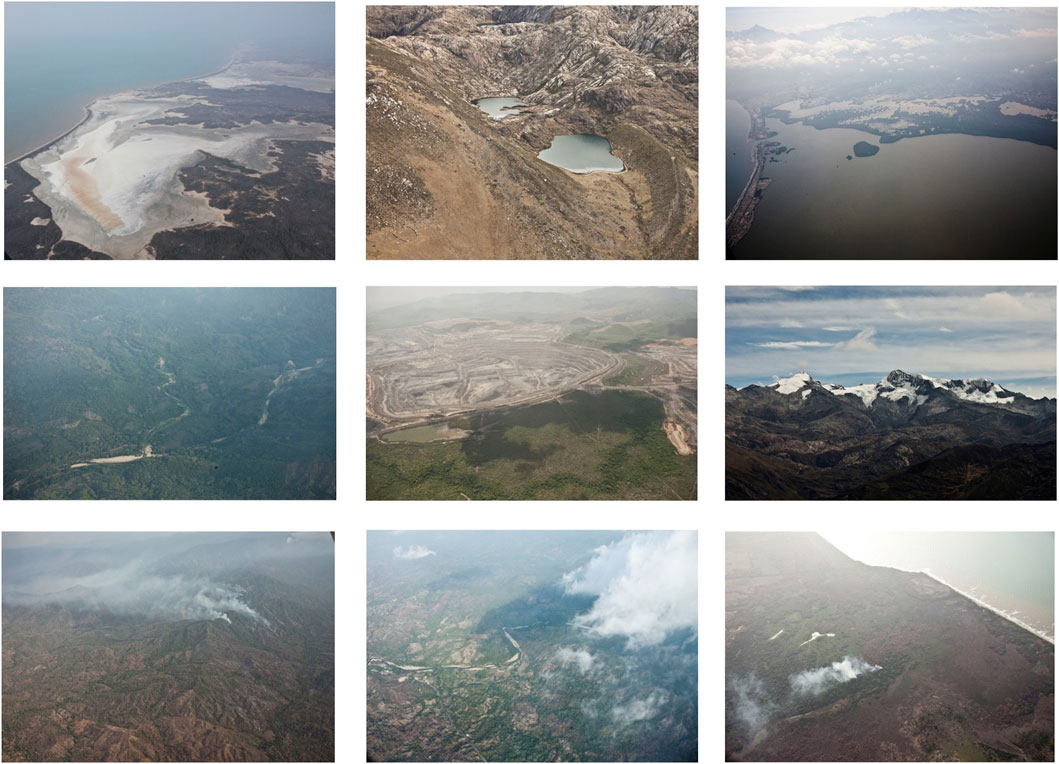
Figure 9. Aerial photographs from the Sierra Nevada de Santa Marta (SNSM). The first two rows highlight geodiversity, showcasing key geological features, while the last row illustrates deforestation caused by land use changes activities. Credits EAFIT University.
Global Comparison of Conflict Zones and Their Impact on Geodiversity
The SNSM is not unique in the challenges it faces from social conflicts, land-use changes, and environmental degradation. Around the world, similar regions have experienced detrimental impacts on their geodiversity and biodiversity due to conflicts, armed groups, and unsustainable land management. These examples from global conflict zones offer a broader perspective on how violence and instability can devastate fragile ecosystems and natural landscapes, further highlighting the urgency for effective conservation efforts.
Democratic Republic of Congo – Virunga National Park (VNP): Famous for its large primates (mountain gorilla, chimpanzees, and Grauer’s gorillas) and variety topography and natural habitats that make it one of the most biodiverse areas in Africa, the VNP, has been severely impacted by decades of armed conflict (Verweijen and Marijnen, 2016). The presence of various militias, coupled with illegal activities like mining, logging and hunting, has devastated the region’s geodiversity. Similar to the SNSM, where illicit crops contribute to deforestation, Virunga has suffered from illegal resource extraction, leading to habitat destruction and soil degradation. The fragile balance between conservation and conflict in Virunga underscores how armed groups can devastate geodiversity through unsustainable practices (Büscher and Davidov, 2013).
Afghanistan – Hindu Kush Mountains (HKM): The ongoing conflict in Afghanistan, particularly in the Hindu Kush Mountain range, has resulted in severe environmental degradation. The pressures of war, overgrazing, deforestation, and unsustainable agriculture have negatively impacted the region’s geodiversity, much like the SNSM, despite the lesser geologic (topographic, geomorphic, lithologies, structural), biotic and climatic diversity of the HKM. Soil erosion, reduced fertility, and the loss of vegetation have all contributed to the decline of biodiversity and ecosystem health. The parallel between these two mountainous regions illustrates how prolonged instability can trigger land-use changes that degrade geodiversity (Saba, 2001).
Middle East – Syrian Civil War: The Syrian Civil War has caused significant environmental damage, with water management systems heavily disrupted by conflict. Desertification and soil degradation, exacerbated by deforestation and unsustainable agricultural expansion, are key issues, similar to the altered water management and soil degradation seen in the SNSM. The destruction of critical infrastructure in Syria has also led to increased vulnerability to natural disasters, paralleling the threats facing geodiversity in conflict-affected regions like the SNSM (Gleick, P. H., 2014).
Sudan – Darfur Conflict: The Darfur conflict in Sudan, driven by competition for natural resources, has caused widespread land degradation and deforestation. Overgrazing, unsustainable agricultural expansion, and the destruction of traditional land-use practices have accelerated desertification, eroding the geodiversity of the region (De Juan, 2015). Like the SNSM, where indigenous practices have been disrupted, Darfur has seen its traditional ecological systems replaced by more harmful methods, further damaging the landscape (Suliman, 1999).
The parallels between these global conflict zones and the SNSM highlight a common pattern: social conflicts, particularly those involving armed groups and illicit activities, directly contribute to the degradation of geodiversity and biodiversity. The examples from Virunga, the Hindu Kush, Syria, and Darfur underscore the broader need for multifaceted solutions that address both social and environmental issues. Effective governance, strong legal frameworks, and the protection of indigenous practices are essential components in mitigating these destructive forces and preserving geodiversity worldwide.
Geodiversity as Important as Biodiversity
Geodiversity is considered as important as biodiversity because, in the end, it underpins biodiversity. Many cultural elements, practices, and traditions express direct links with geodiversity. “Geodiversity encompasses all the parts of nature that are not alive, including everything from minerals and fossils to soils and spectacular landscapes. Scientific knowledge about how geological and geomorphological processes occur in nature is extremely important for disaster risk prevention (earthquakes, tsunamis, volcanoes, flooding, and landslides) and to support smart solutions in land use planning and spatial management. Geodiversity acts as a natural laboratory and textbook, teaching new generations about Earth’s history, the sustainable use of Earth’s resources, and the science necessary to overcome the challenges of tomorrow” (Geodiversity Day, 2022).
Geoconservation aims to identify and protect heritage related to Earth Sciences (geoheritage) to prevent its loss and to exploit its pedagogic potential. However, maintaining the integrity of geoheritage is increasingly complex for decision-makers who must balance economic growth and social responsibilities (Henriques et al., 2011). Geological resources provide raw materials essential for people’s wellbeing and economic development, but most of these resources are non-renewable, and the extraction methods often deployed are deleterious to the environment and the communities. Consequently, valuable geological and geomorphological features, processes, sites, and specimens considered as geoheritage are particularly vulnerable. Once damaged, they are lost for future generations, taking away part of the planet’s history with them (Brocx and Semeniuk, 2015). Understanding the Earth’s past helps society address many of today’s environmental problems and find solutions to mitigate them in the present and future. Hence, the protection of geological heritage is crucial, along with enhancing awareness and disseminating geoscientific knowledge to society (Carreras and Druguet, 2000).
Although various conservation figures with different objectives coexist in the SNSM (e.g., UNESCO’s Biosphere Reserve under the Man and Biosphere Program, 19 indigenous reserves, and two National Natural Parks), socio-environmental conflict is evident in many aspects, arising from both legal and illegal phenomena. Notably, there are ongoing territorial disputes among armed groups and issues related to illicit drug production and trafficking, as well as the development of 152 mining operations concentrated along the edges of the Sierra. One of the most significant conflicts in the SNSM takes place between local communities, mostly indigenous, and mining operations due to the degradation of the territory caused by the latter. Exploration and exploitation of geological resources (metals, energy, strategic) often trigger socio-economic and socio-environmental conflict (Pyhälä, 2020). The concept of geodiversity surpasses the often-dominant extractivist approach in geology, advocating for new uses of the geological discipline as opportunities for more sustainable development consolidate. Implementing adequate environmental policies in the SNSM is imperative for holistic development, considering the needs of both the environment and the communities.
The SNSM is, in essence, a territory of indigenous communities. Understanding the relationship between these communities and their territory is essential. The different communities in the SNSM have repeatedly been forced to migrate internally, losing their roots and ancestral land, and being compelled to abandon traditional activities. As Yeismith Armeta Ama, coordinator of the historical memory process of the Wiwa peoples, stated: “The armed conflict has violated, mistreated, and murdered our sacred territories. This violence has affected our spiritual fathers who live in the water, the trees, the plants” (Centro de Memoria Histórica, 2015). Due to the exchange of knowledge between national and indigenous governments, the idea of the territory as a victim of conflict has emerged and been incorporated into the Decree Law of Victims (4633-2022, Arts. 3, 8, 45). This recognition acknowledges the diversity of epistemologies and relationships between indigenous peoples and Mother Earth, “promoting a certain recognition of the increasingly porous borders between the realms of the given or natural (kosmos) and the constructed or cultural (politikos), and an opportunity to highlight the place from which the State regulates the relations between the public and the private, between the universal and the particular, between science and belief” (Ruiz-Serna, 2017). Considering Ruiz-Serna’s analysis (2017), does the concept of territory as a victim imply the equal importance of considering the living and non-living components affected by the armed conflict? If so, does it legitimate the necessity of including geodiversity as part of the territory in the implementation of the “Victims’ Law”?
Indigenous peoples have long modified and used geological heritage in harmony with the landscape and its ecosystems for their prosperity and survival. The landscape and many of its natural components are deeply ingrained in their culture and often are integral parts of their cosmogonies. In doing so, they have enriched the modern landscape and inherited a geodiversity with extensive knowledge of their environments and the importance of their conservation. According to Worton (2008), it is essential to consider indigenous knowledge and practices within modern definitions of geoconservation. The interaction of knowledge between scientists, experts, and communities (including indigenous, Afro-descendants, peasants, farmers, and other inhabitants) is crucial to co-create ideas that could be proposed and adopted by the government. Given the complexity of the Colombian socio-political context, especially in the SNSM, the first step towards real co-creation is reconciliation between actors and with the territory. According to Slaghekke (2020), this reconciliation is characterized by ambivalent conceptions that perpetuate violent dynamics.
Colonial conceptions of nature were imposed on Indigenous peoples to establish unequal power relationships (Adnan, 2004; Escobar, 2010; Mukherjee, 2010). These conceptions persisted during neo-colonial periods, with unfair forest projects around the world pushing these communities to prioritize development using modern technologies over their traditions. For indigenous peoples, the Earth is not a fixed entity; it is an active actor that can influence their lives, actions, and ways of being. They rely on spirituality and experiences to set up connections among the different actors of nature (Datta, 2015). In Colombia, the Laws “de Origen” (or Law Sé, ancestral indigenous law) and “Línea Negra” (Resolution No. 000002 of 1973) are legal mechanisms employed by indigenous communities for the organization of their territories and their respective spiritual connection with them (Cárdenas, 2002). In this context, the “mamos,” who are the spiritual leaders of the SNSM, use their ethnobiological knowledge to guide the ordering, management, and handling of these territories (Barbosa, 2011). These laws also encompass the relationship between sacred places and territories (Rodríguez-Osorio, 2014) to mitigate the environmental and cultural damages caused by the profanation of sacred territories of native people (Kogui, Wiwa, Kankuamo, and Arhuaco) and the indiscriminate use of natural resources (Duque, 2009).
In this context, it is crucial to begin by defining a clearer and more participatory governance structure for the SNSM that allows different actors to take part securely. This is particularly important in Colombia, where conservation initiatives are often neglected by authorities, influenced by illegal groups, or threatened by various challenges. Defending the environment and territories has unfortunately led to the deaths of many environmental and social leaders. Viloria-de-la-Hoz (2005) points out inadequate administration of the Sierra, hindering agile and effective territorial management. This is partly due to the overlapping responsibilities of several entities, including the three departmental administrations (Guajira, Magdalena, and Cesar), 20 municipalities, three regional autonomous corporations (CORPAMAG, CORPOCESAR, and CORPOGUAJIRA), two national parks, the Institute of Anthropology and History (ICANH), among others. This overlapping jurisdiction is exacerbated by the overlapping of titles, responsibilities, and mining rights, which is inconsistent with the goals and purposes of environmental entities.
According to Viloria-de-la-Hoz (2005), Sierra and its stakeholders cannot work as a team without a unified plan. Having a guiding plan and a sole entity to follow up on it is imperative. The lack of concerted environmental planning and the deficiencies in environmental administration have contributed to the degradation of the SNSM. Consequently, there is an urgent need to define and implement clear, effective policies and plans to protect and manage the geodiversity and biodiversity of the SNSM, ensuring the sustainable development of the region.
Conclusion
Diverse narratives about the SNSM unveil a concerning tale of environmental degradation intricately woven with the threads of social conflicts, historical events, and unsustainable human activities. The assessment of this unique heritage spotlights the urgent need for intervention and conservation efforts to safeguard the rich geodiversity and biodiversity of the Sierra.
The significant threats identified include the loss of biodiversity hotspots, altered water management, soil degradation, reduction of slope stability, fragmentation of ecological corridors, and erosion of indigenous knowledge. Historical events, such as the “Bonanza Marimbera” and “Bonanza Coquera,” underscore the nexus between armed conflict, illicit activities, and environmental degradation, emphasizing the complex interplay of social, economic, and ecological factors. Statistical insights reveal alarming trends, with peak deforestation in 2017 and 2003, predominantly fueled by illicit crops, illegal wood trade, and unchecked agricultural expansion. Or findings also highlight the consequences of weak presence by governmental entities, allowing the prevalence of illegal groups in controlling the territory and amplifying the environmental-conservation challenges faced by the SNSM.
The Sierra, a UNESCO-declared Biosphere Reserve, stands at the nexus of orogenic processes shaping the Northern Andes. Yet, increasing socio-environmental conflicts, manifesting as changes in land use, deforestation, and agricultural expansion, have catalyzed a cascade of detrimental effects on geodiversity and hence on biodiversity. Traditional land management practices, crucial for supporting geodiversity, face disruption, further worsening the challenges. This complex context demands innovation in the approaches for environmental management strategies and actions. Strengthening governance structures, enhancing law enforcement, and promoting community engagement in conservation in initiatives are crucial steps.
Addressing these issues requires a multifaceted approach that prioritizes the protection of both living and non-living components of the environment. By empowering local communities and recognizing their traditional land management practices, we can foster a sense of ownership and stewardship over the region’s natural resources. Furthermore, there is a need to shift the paradigm of resource use and governance. Geological resources should be seen not merely as materials for extraction but as integral parts of the region’s heritage that can contribute to sustainable development. Implementing innovative environmental policies that balance economic growth with ecological preservation will be essential in safeguarding the SNSM for future generations.
Data Availability Statement
The raw data supporting the conclusions of this article will be made available by the authors, without undue reservation.
Author Contributions
JZ-H was the principal investigator, MM-C contributed to the construction of geodiversity models, and CG-T provided expertise on the role of geoconservation in Sierra Nevada de Santa Marta. All authors contributed to the article and approved the submitted version. SARM Contributed to the improvement and updating of the original text on all sections, especially on structure and content.
Funding
The author(s) declare financial support was received for the research, authorship, and/or publication of this article. MM-C acknowledges the support from the “Fellowship on Science Diplomacy” grant funded by MinCiencias 2023–2024 and to EAFIT University for providing sabbatical support.
Conflict of Interest
The authors declare that the research was conducted in the absence of any commercial or financial relationships that could be construed as a potential conflict of interest.
Publisher’s Note
All claims expressed in this article are solely those of the authors and do not necessarily represent those of their affiliated organizations, or those of the publisher, the editors and the reviewers. Any product that may be evaluated in this article, or claim that may be made by its manufacturer, is not guaranteed or endorsed by the publisher.
Acknowledgments
The authors also wish to express special thanks to the reviewers for their valuable comments, which significantly improved the original manuscript.
Footnotes
1https://www.ipcc.ch/report/ar6/syr/downloads/report/IPCC_AR6_SYR_LongerReport.pdf
2http://www.ideam.gov.co/web/ecosistemas/glaciares-colombia
3IGAC from https://www.colombiaenmapas.gov.co/?e=-82.43784778320864,-0.17644239911865092,-71.23179309571162,9.90326984502256,4686&b=igac&u =0&t=23&servicio=205
4https://www.unesco.org/en/mab/sierra-nevada-de-santa-marta
References
Adnan, S. (2004). Migration, Land Alienation and Ethnic Conflict: Causes of Poverty in the Chittagong Hill Tracts of Bangladesh. Dhaka, Bangladesh: Research and Advisory Services. Available at: https://lccn.loc.gov/2004329932 (Accessed October 4, 2024).
Alvarado-Villalon, F., Harrison, D. J., and Steadman, E. J. (2003). Alluvial Mining of Aggregates in Costa Rica. Available at: https://nora.nerc.ac.uk/id/eprint/527305.
Álvarez, M. D. (2007). “Environmental Damage From Illicit Drug Crops in Colombia,” in Extreme Conflict and Tropical Forests. World Forests. Editors W. D. Jong, D. Donovan, and K. I. Abe (Dordrecht: Springer), 5, 133–147. doi:10.1007/978-1-4020-5462-4_8
Antonelli, A., Kissling, W. D., Flantua, S. G., Bermúdez, M. A., Mulch, A., Muellner-Riehl, A. N., et al. (2018). Geological and Climatic Influences on Mountain Biodiversity. Nat. Geosci. 11 (10), 718–725. doi:10.1038/s41561-018-0236-z
Antrop, M. (2006). Sustainable Landscapes: Contradiction, Fiction, or Utopia? Landsc. UrbanPlan. 75, 187–197. doi:10.1016/j.landurbplan.2005.02.014
Argyriou, A. V., Sarris, A., and Teeuw, R. M. (2016). Using Geoinformatics and Geomorphometrics to Quantify the Geodiversity of Crete, Greece. Int. J. Appl. Earth Observation Geoinformation 51, 47–59. doi:10.1016/j.jag.2016.04.006
Arias, M. A., Ibánez, A. M., and Zambrano, A. (2014). Agricultural Production Amid Conflict: The Effects of Shocks, Uncertainty, and Governance of Non-State Armed Actors. Tech. Rep. Available at: http://hdl.handle.net/1992/8452 (Accessed October 16, 2023).
Arias-Díaz, A., Murcia, H., Vallejo-Hincapié, F., and Németh, K. (2023). Understanding Geodiversity for Sustainable Development in the Chinchiná River Basin, Caldas, Colombia. Land 12 (11), 2053. doi:10.3390/land12112053
Armenteras, D., Cabrera, E., Rodríguez, N., and Retana, J. (2013). National and Regional Determinants of Tropical Deforestation in Colombia. Reg. Environ. Change 13, 1181–1193. doi:10.1007/s10113-013-0433-7
Badgley, C., Smiley, T. M., Terry, R., Davis, E. B., DeSantis, L. R., Fox, D. L., et al. (2017). Biodiversity and Topographic Complexity: Modern and Geohistorical Perspectives. Trends Ecol. Evol. 32 (3), 211–226. Available at: https://www.cell.com/trends/ecology-evolution/abstract/S0169-5347(16)30244-0 (Accessed April 20, 2024).
Ballesteros-Cánovas, J. A., Stoffel, M., Martín-Duque, J. F., Corona, C., Lucía, A., Bodoque, J. M., et al. (2017). Gully Evolution and Geomorphic Adjustments of Badlands to Reforestation. Sci. Rep. 7 (1), 45027. doi:10.1038/srep45027
Balslev, H., and Luteyn, J. (1992). Páramo: An Andean Ecosystem Under Human Influence. London: Academic Press. Available at: https://www.scopus.com/record/display.uri?eid=2-s2.085040958239&origin=inward&txGid=b60b30e95cc4afd569552d4d364370ac (Accessed October 6, 2023).
Barbosa, R. (2011). El Orden del Todo: Sierra Goanawindwa-Shwndwa un Territorio de Memorias, Tendencias y Tensiones en Torno al Ordenamiento Ancestral. IEPRI, 1. Medellín: Universidad Nacional de Colombia, 242. Available at: https://www.scribd.com/document/378088964/Orden-del-todo-final-def-pmd-1-pdf (Accessed March 3, 2024).
Bayona, G., Rapalini, A., and Costanzo-Alvarez, V. (2006). Paleomagnetism in Mesozoic Rocks of the Northern Andes and Its Implications in Mesozoic Tectonics of Northwestern South America. Earth Planets Space 58, 1255–1272. doi:10.1186/BF03352621
Britto, L. (2020). Marijuana Boom: The Rise and Fall of Colombia’s First Drug Paradise. Oakland: Univ of California Press. Available at: https://books.google.com.co/books?hl=es&lr=&id=xX7JDwAAQBAJ&oi=fnd&pg=PR11&dq=Britto,+Lina.%C2%A0Marijuana+Boom:+The+Rise+and+fall+of+Colombia%27s+first+drug+paradise.+Univ+of+California+Press,+2020.&ots=Jg38yOpFyB&sig=wPEuZuwcZxPQyogJDhql97h36tY&redir_esc=y#v=onepage&q=Britto%2C%20Lina.%C2%A0Marijuana%20Boom%3A%20The%20Rise%20and%20fall%20of%20Colombia's%20first%20drug%20paradise.%20Univ%20of%20California%20Press%2C%202020.&f=false (Accessed May 15, 2023).
Brocx, M., and Semeniuk, V. (2015). “Geology: From Antiquity to Modern Day Geoheritage and Geoconservation, With Britain as a Case Study,” in From Geoheritage to Geoparks: Case Studies From Africa and Beyond. Editors E. Errami, M. Brocx, and V. Semeniuk (Cham: Springer), 35–53. doi:10.1007/978-3-319-10708-0#page=42
Büscher, B., and Davidov, V. (2013). The Ecotourism-Extraction Nexus: Political Economies and Rural Realities of (Un)comfortable Bedfellows. London: Routledge. ISBN: 978-0-415-82429-9.
Bustamante, C., Cardona, A., Saldarriaga, M., García-Casco, A., Valencia, V., and Weber, M. (2009). Metamorfismo de los Esquistos Verdes y Anfibolitas Pertenecientes a los Esquistos de Santa Marta, Sierra Nevada de Santa Marta (Colombia): ¿Registro de la Colisión Entre el Arco Caribe y la Margen Suramericana? Bol. Ciencias la Tierra (25), 07–25. Available at: http://www.scielo.org.co/scielo.php?pid=S0120-36302009000100002&script=sci_arttext.
Calderon-Luna, F. A., and Torres-Guisao, Y. A. (2017). Implicaciones ambientales y sociales de las fumigaciones aéreas con glifosato en Colombia: el ejemplo de la Sierra Nevada de Santa Marta 2002–2006. Medellín: UNAULA. Available at: http://repositorio.unaula.edu.co:4000/items/f565fdf4-c0eb-47c9-9f22-d2e822c2c257.
Carbonó, E., and Lozano-Contreras, G. (1997). Endemismos y Otras Singularidades de la Sierra Nevada de Santa Marta, Colombia. Posibles Causas de Origen y Necesidad de Conservarlos. Rev. la Acad. Colomb. Ciencias Exactas, Físicas Nat. 21 (81), 409–419. Available at: https://scholar.google.com/scholar_lookup?title=Endemismos%20y%20otras%20singularidades%20de%20la%20Sierra%20Nevada%20de%20Santa%20Marta%2C%20Colombia.%20Posibles%20causas%20de%20origen%20y%20necesidad%20de%20conservarlos&ublication_year=1997&uthor=E.%20Carbon%C3%B3&uthor=G.%20Lozano-Contreras.
Cárdenas, F. (2002). Antropología y Ambiente: Enfoques para una Comprensión de la Relación Ecosistema-Cultura. Bogotá: Pontificia Universidad Javeriana. Available at: https://books.google.co.in/books/about/Antropolog%C3%ADa_y_ambiente.html?id=SfqqtJVbzwAC&redir_esc=y (Accessed May 10, 2024).
Cardona, A., Duque, J., Ruiz, J., Valencia, V., Bayona, G., Jaramillo, C., et al. (2008a). Geochronology and Tectonic Implications of Granitoids Rocks From the Northwestern Sierra Nevada de Santa Marta and Surrounding Basins, Northeastern Colombia: Late Cretaceous to Paleogene Convergence, Accretion, and Subduction Interactions Between the Caribbean and South American plates. 18va Conf. Geol. del Caribe. República Dominic.
Cardona, A., Garcia-Casco, A., Ruiz, J., Valencia, V., Bustamante, C., Garzón, A., et al. (2008b). Late Cretaceous Caribbean-South America Interactions: Insights From the Metamorphic Record of the NW Sierra Nevada de Santa Marta, Colombia. 18va Conf. Geol. del Caribe. República Dominic.
Carreras, J., and Druguet, E. (2000). “Geological Heritage, an Essential Part of the Integral Management of World Heritage in Protected Sites,” in Geological Heritage: Its Conservation and Management. III International Symposium ProGEO on the Conservation of the Geological Heritage, 95–110. Available at: https://books.google.com.co/books?hl=es&lr=&id=fGAtSnF3n_QC&oi=fnd&pg=PA95&dq=Carreras,+J.,+%26+Druguet,+E.+(2000).+Geological+heritage,+an+essential+part+of+the+integral+management+of+World+heritage+in+protected+sites.+In%C2%A0Geological+heritage:+Its+conservation+and+management.+III+International+Symposium+ProGEO+on+the+conservation+of+the+geological+heritage%C2%A0(pp.+95-110).&ots=IbFT4CcY_s&sig=569nhXJ8ILBJI2ArfkEZZX2SrVc&redir_esc=y#v=onepage&q&f=false (Accessed September 20, 2024).
Cavelier, J., Aide, T., Santos, C., Eusse, A., and Dupuy, J. (1998). The Savannization of Moist Forests in the Sierra Nevada de Santa Marta, Colombia. J. Biogeogr. 25 (5), 901–912. doi:10.1046/j.1365-2699.1998.00222.x
Cediel, F. (2019). “Phanerozoic Orogens of Northwestern South America: Cordilleran-Type Orogens. Taphrogenic Tectonics. The Maracaibo Orogenic Float. The Chocó-Panamá Indenter,” in Geology and Tectonics of Northwestern South America: The Pacific-Caribbean-Andean Junction. Bogotá: Springer Cham, 3–95. doi:10.1007/978-3-319-76132-9_1
Cediel, F., Shaw, R. P., and Cáceres, C. (2003). “Tectonic Assembly of the Northern Andean Block,” in The Circum-Gulf of Mexico and the Caribbean: Hydrocarbon Habitats, Basin Formationand Plate Tectonics, Formation, and Plate Tectonics (Bogotá: AAPG Memoir), 79, 815–848. doi:10.1306/M79877C37
Centro de Memoria Histórica (CMH) (2015). Indígenas se Reúnen en la Sierra Nevada por sus Víctimas y la Memoria. Available at: http://www.centrodememo-riahistorica.gov.co/noticias/noticias-cmh/indigenas-se-reunen-en-la-sierra-nevada-por-sus-victimas-y-la-memoria (Accessed September 21, 2024).
Centro Nacional de Memoria Histórica (CNMH) (2015). Indígenas se Reúnen en la Sierra Nevada por sus Víctimas y la Memoria. New York: CNMH. Available at: https://centrodememoriahistorica.gov.co/indigenas-se-reunen-en-la-sierra-nevada-por-sus-victimas-y-la-memoria/ (Accessed March 20, 2024).
Colmenares, F., Mesa, A., Roncancio, J., Arciniegas, E., Pedraza, P., Cardona, A., et al. (2007). Geología de las Planchas 11, 12, 13, 14, 18, 19, 20, 21, 25, 26, 27, 33, 34 y 40. Proyecto: Evolución Geohistórica de la Sierra Nevada de Santa Marta. Available at: https://srvags.sgc.gov.co/Flexviewer/Estado_Cartografia_Geologica/ (Accessed April 12, 2024).
Comer, P. J., Pressey, R. L., Hunter, M. L., Schloss, C. A., Buttrick, S. C., Heller, N., et al. (2015). Incorporating Geodiversity Into Conservation Decisions. Conserv. Biol. 29 (3), 692–701. doi:10.1111/cobi.12508
Commoner, B. (1990). Making Peace With the Planet. Bogotá: The New Press, 304. Available at: https://lccn.loc.gov/89043241 (Accessed May 16, 2024).
Corrales, L. M. G., Avila, H., and Gutierrez, R. R. (2019). Land-Use and Socioeconomic Changes Related to Armed Conflicts: A Colombian Regional Case Study. Environ. Sci. Policy 97, 116–124. doi:10.1016/j.envsci.2019.04.012
Crutzen, P. J. (2016). “Geology of Mankind,” in Paul J. Crutzen: A Pioneer on Atmospheric Chemistry and Climate Change in the Anthropocene. SpringerBriefs on Pioneers in Science and Practice. Editors P. Crutzen, and H. Brauch (Cham: Springer), 50, 211–215. doi:10.1007/978-3-319-27460-7_10
Cuatrecasas, J. (1968). “Páramo Vegetation and Its Life Forms,” in Geoecology of Mountainous Regions of Tropical America. Editor C. Troll (Bonn: Coll. Geogr.), 9, 163–186. Available at: https://scholar.google.com/scholar_lookup?&title=P%C3%A1ramo%20vegetation%20and%20its%20life-forms&pages=163-186&publication_year=1968&author=Cuatrecasas%2CJ (Accessed February 2, 2024).
Culbert, P. D., Radeloff, V. C., St-Louis, V., Flather, C. H., Rittenhouse, C. D., Albright, T. P., et al. (2012). Modeling Broad-Scale Patterns of Avian Species Richness Across the Midwestern United States With Measures of Satellite Image Texture. Remote Sens. Environ. 118, 140–150. doi:10.1016/j.rse.2011.11.004
Datta, R. (2015). A Relational Theoretical Framework and Meanings of Land, Nature, and Sustainability for Research With Indigenous Communities. Local Environ. 20 (1), 102–113. doi:10.1080/13549839.2013.818957
DeFries, R. (2008). Terrestrial Vegetation in the Coupled Human-Earth System: Contributions of Remote Sensing. Annu. Rev. Environ. Resour. 33 (1), 369–390. doi:10.1146/annurev.environ.33.020107.113339
De Juan, A. (2015). Long-term Environmental Change and Geographical Patterns of Violence in Darfur, 2003–2005. Polit. Geogr. 45, 22–33. doi:10.1016/j.polgeo.2014.09.001
De la Paix, M. J., Lanhai, L., Xi, C., Ahmed, S., and Varenyam, A. (2013). Soil Degradation and Altered Flood Risk as a Consequence of Deforestation. Land Degrad. Dev. 24 (5), 478–485. doi:10.1002/ldr.1147
Díaz-Escobar, A., and Sánchez-Torres, F. (2004). A Geography of Illicit Crops (Coca Leaf) and Armed Conflict in Colombia. Medellín: Universidad de los Andes, Facultad de Economía, CEDE. Available at: http://hdl.handle.net/1992/7873 (Accessed March 11, 2024).
Duque, P. (2009). “Lo Sagrado Como Argumento Jurisdiccional en Colombia,” in La Reclamación de Tierras Indígenas Como Argumento de Autonomía Cultural en la Sierra Nevada de Santa Marta. Bogotá: Universidad Nacional de Colombia. Available at: https://repositorio.unal.edu.co/handle/unal/70253 (Accessed November 10, 2023).
Duque-Trujillo, J. F., Orozco-Esquivel, T., Sánchez, C. J., and Cárdenas-Rozo, A. L. (2019). “Paleogene Magmatism of the Maracaibo Block and Its Tectonic Significance,” in Geology and Tectonics of Northwestern South America. Editors F. Cediel, and R. P. Shaw (Cham: Springer). doi:10.1007/978-3-319-76132-9_7
Durán-Izquierdo, M., and Olivero-Verbel, J. (2021). Vulnerability Assessment of Sierra Nevada de Santa Marta, Colombia: World’s Most Irreplaceable Nature Reserve. Glob. Ecol. Conservation 28, e01592. doi:10.1016/j.gecco.2021.e01592
Escobar, A. (2010). Latin America at a Crossroads. Cult. Stud. 24 (1), 1–65. doi:10.1080/09502380903424208
Faria, D., Morante-Filho, J., Baumgarten, J., Bovendorp, R., Cazetta, E., Gaiotto, F., et al. (2023). The Breakdown of Ecosystem Functionality Driven by Deforestation in a Global Biodiversity Hotspot. Biol. Conserv. 283, 110126. doi:10.1016/j.biocon.2023.110126
Fischlin, A., Midgley, G. F., Price, J. T., Leemans, R., Gopal, B., Turley, C., et al. (2007). “Ecosystems, Their Properties, Goods and Services,” in Climate Change 2007: Impacts, Adaptation and Vulnerability. Contribution of Working Group II to the Fourth Assessment Report of the Intergovernmental Panel of Climate Change (IPCC). Editors M. L. Parry, O. F. Canziani, J. P. Palutikof, P. J. van der Linden, and C. E. Hanson (Cambridge, UK: Cambridge University Press), 211–272. Available at: https://www.ipcc.ch/report/ar4/wg2/.
Fortier, R. P., Bernal-Escobar, M., Kullberg, A. T., Osorio Ospina, J. C., Herrera Medina, Y., Aragón, L., et al. (2024). Diversity, composition, and structure of a 1-hectare tree plot in the cloud forest of the Sierra Nevada de Santa Marta, Colombia. Ann. For. Sci. 81 (1), 24. doi:10.1186/s13595-024-01239-6
Funari, P. (2023). Las Ciudades Olvidadas: Las Sociedades Originarias de la Sierra Nevada de Santa Marta Ocupación Espacial y Desarrollo Arquitectónico, Siglos X al XVI. Santa Marta, Madrid: Ediciones Doce Calles, Universidad Pablo de Olavide, Área de Historia de América, Instituto Colombiano de Arqueología e Historia, Universidad del Magdalena, 416. ISBN: 978-84-9744-269-5.
Gadgil, M. (1987). Social Restraints on Exploiting Nature: The Indian Experience. Dev. seeds change 1, 26–30. Available at: https://wgbis.ces.iisc.ac.in/biodiversity/pubs/mg/pdfs/mg063.pdf.
Gleick, P. H. (2014). Water, Drought, Climate Change, and Conflict in Syria. Weather, Clim. Soc. 6 (3), 331–340. doi:10.1175/WCAS-D-13-00059.1
González-González, A., Villegas, J. C., Clerici, N., and Salazar, J. F. (2021). Spatial-Temporal Dynamics of Deforestation and Its Drivers Indicate Need for Locally-Adapted Environmental Governance in Colombia. Ecol. Indic. 126, 107695. doi:10.1016/j.ecolind.2021.107695
Gordon, J. E. (2018). Mountain Geodiversity: Characteristics, Values and Climate Change. Mt. Clim. Biodivers., 137–154. Available at: https://books.google.com.co/books?hl=es&lr=&id=V5JRDwAAQBAJ&oi=fnd&pg=PA137&dq=Gordon,+J.+E.+(2018).+Mountain+geodiversity:+Characteristics,+values+and+climate+change.+Mountains,+climate,+and+biodiversity,+137-154&ots=FCPJmkRVao&sig=CD6y3VXl1qmywe-3CpI2aCGJkZE&redir_esc=y#v=onepage&q&f=false.
Goyes, D., South, N., Abaibira, M., Baicué, P., Cuchimba, A., and Ñeñetofe, D. (2021). Genocide and Ecocide in Four Colombian Indigenous Communities: The Erosion of a Way of Life and Memory. Br. J. Criminol. 61 (4), 965–984. doi:10.1093/bjc/azaa109
Gray, M. (2004). Geodiversity: Valuing and ConservingAbiotic Nature. Chichester: Wiley. ISBN: 978-0-470-74215-0.
Gray, M. (2005). Geodiversity and Geoconservation: What, Why, and How? George Wright Society. George Wright Forum 22 (3), 4–12. Available at: https://www.jstor.org/stable/43597951.
Gray, M. (2013). Geodiversity: Valuing and Conserving Abiotic Nature. 2nd ed. Chichester: Wiley Blackwell. ISBN: 978-0-470-74215-0.
Gray, M. (2019). Geodiversity, Geoheritage and Geoconservation for Society. Int. J. Geoheritage Parks 7 (4), 226–236. doi:10.1016/j.ijgeop.2019.11.001
Gutiérrez-Martínez, L. (2021). “Estimación de la Geodiversidad en la Cuenca del rio Palomino-Parque Nacional Natural Sierra Nevada de Santa Marta, Como Herramienta para la Gestión Integral del Territorio. Bachelor’s Thesis. Bogotá: Universidad EAFIT. Available at: http://hdl.handle.net/10784/30588 (Accessed October 9, 2023).
Gutiérrez-Montoya, N. (2022). Las Ciudades Olvidadas. Las Sociedades Originarias de la Sierra Nevada de Santa Marta, Siglos X al XVI. Aranjuez: Ediciones Doce Calles, Universidad Pablo de Olavide, Instituto Colombiano de Antropología e Historia, Universidad del Magdalena, 413. ISBN: 9789587466058.
Gutiérrez-Villegas, H. F. (2024). Procesos de desterritorializacion y reterritorializacion en la sierra nevada de santa marta (1980–2010). Bogotá: Universidad Pedagógica. Available at: http://repository.pedagogica.edu.co/handle/20.500.12209/20209.
Haines-Young, R. (2009). Land Use and Biodiversity Relationships. Land use policy 26, S178–S186. doi:10.1016/j.landusepol.2009.08.009
Hansen, M. C., Potapov, P. V., Moore, R., Hancher, M., Turubanova, S. A., Tyukavina, A., et al. (2013). High-Resolution Global Maps of 21st-Century Forest Cover Change. Science 342 (15 November), 850–853. doi:10.1126/science.1244693
Henao, M. V., and Molina, E. H. (2010). Los Daños Ambientales y Socioculturales en la Sierra Nevada de Santa Marta y Falta de Protección Efectiva. London; Observatorio de Territorios Étnicos y Campesinos. Available at: https://etnoterritorios.org/apc-aa-files/92335f7b3cf47708a7c984a309402be7/sierra-nevada-helo-y-guerrero-corr-jav-2-1-.pdf (Accessed September 4, 2024).
Henriques, M. H., dos Reis, R. P., Brilha, J., and Mota, T. (2011). Geoconservation as an Emerging Geoscience. Geoheritage 3, 117–128. doi:10.1007/s12371-011-0039-8
Hofstede, R., Segarra, P., and Mena Vásconez, P. (2003). Los Páramos del Mundo. Proyecto Atlas Mundial de los Páramos. Quito: Global Peatland Initiative/NC-IUCN/EcoCiencia. Available at: https://hdl.handle.net/11245/1.213878.
Holdridge, L. R. (1967). Life Zone Ecology. San Jose, Costa Rica: Tropical Science Center, 206. doi:10.1180/minmag.1967.036.280.18
Hooghiemstra, H., and Flantua, S. G. A. (2019). “Colombia in the Quaternary: An Overview of Environmental and Climatic Change,” in The Geology of Colombia Book. Editor J. Gómez Chapter 2 (Bogotá: Servicio Geológico Colombiano, Quaternary), 4, 33–57. Available at: https://hdl.handle.net/11245.1/a0e6d722-8bb8-427a-9e91-39d5d907b4c7.
Houghton, R. A. (1994). The Worldwide Extent of Land-Use Change. BioScience 44, 305–313. doi:10.2307/1312380
Huneeus, A., and Rueda-Sáiz, P. (2021). Territory as a Victim of Armed Conflict. Int. J. Transitional Justice 15 (1), 210–229. doi:10.1093/ijtj/ijab002
Idárraga-García, J., and Romero, J. (2010). Neotectonic Study of the Santa Marta Fault System, Western Foothills of the Sierra Nevada de Santa Marta, Colombia. J. S. Am. Earth Sci. 29 (4), 849–860. doi:10.1016/j.jsames.2009.11.004
IDEAM, I., IAVH, and S., IIAP (2017). Mapa Ecosistemas Continentales, Costeros y Marinos de Colombia (MEC), Escala 1: 100.000. Bogotá (Colombia).
Jha, S., and Bawa, K. S. (2006). Population Growth, Human Development, and Deforestation in Biodiversity Hotspots. Conserv. Biol. 20 (3), 906–912. doi:10.1111/j.1523-1739.2006.00398.x
Jiménez, A., Molina, M. F., and Le Deunff, H. (2015). Indigenous Peoples and Industry Water Users: Mapping the Conflicts Worldwide. Aquat. Procedia 5, 69–80. doi:10.1016/j.aqpro.2015.10.009
Kupfer, J. A., and Franklin, S. B. (2009). Linking Spatial Pattern and Ecological Responses in Human-Modified Landscapes: The Effects of Deforestation and Forest Fragmentation on Biodiversity. Geogr. Compass 3 (4), 1331–1355. doi:10.1111/j.1749-8198.2009.00245.x
Lal, R. (1996). Deforestation and Land-Use Effects on Soil Degradation and Rehabilitation in Western Nigeria. I. Soil Physical and Hydrological Properties. Land Degrad. Dev. 7 (1), 19–45. doi:10.1002/(SICI)1099-145X(199603)7:1<19::AID-LDR212>3.0.CO;2-M
Le Houérou, H. N. (2002). Man-Made Deserts: Desertization Processes and Threats. Arid Land Res. Manag. 16 (1), 1–36. doi:10.1080/153249802753365296
López-Moreno, J., Ceballos, J. L., Rojas-Heredia, F., Zabalza-Martinez, J., Vidaller, I., Revuelto, J., et al. (2020). Topographic Control of Glacier Changes Since the End of the Little Ice Age in the Sierra Nevada de Santa Marta mountains, Colombia. J. S. Am. Earth Sci. 104, 102803. doi:10.1016/j.jsames.2020.102803
Manjarrés-García, G., and Manjarrés-Pinzón, G. (2004). Contribución al Conocimiento Hidrobiológico de la parte Baja de los Ríos de la Vertiente Noroccidental de la Sierra Nevada de Santa Marta, Colombia. Intropica 1 (1), 39–50. doi:10.21676/23897864.451
Marston, R. A. (2010). Geomorphology and Vegetation on Hillslopes: Interactions, Dependencies, and Feedback Loops. Geomorphology 116 (3-4), 206–217. doi:10.1016/j.geomorph.2009.09.028
Martensen, A., Ribeiro, M., Banks-Leite, C., Prado, P., and Metzger, J. (2012). Associations of Forest Cover, Fragment Area, and Connectivity With Neotropical Understory Bird Species Richness and Abundance. Conserv. Biol. 26 (6), 1100–1111. doi:10.1111/j.1523-1739.2012.01940.x
Maslin, M. A., and Lewis, S. L. (2015). Anthropocene: Earth System, Geological, Philosophical and Political Paradigm Shifts. Anthropocene Rev. 2 (2), 108–116. doi:10.1177/2053019615588791
Maunder, M., Leiva, A., Santiago-Valentín, E., Stevenson, D. W., Acevedo-Rodríguez, P., Meerow, A. W., et al. (2008). Plant Conservation in the Caribbean Island Biodiversity Hotspot. Botanical Rev. 74, 197–207. doi:10.1007/s12229-008-9007-7
Mayr, J., Rodriguez, G., Ortiz, N., and Sanchez, H. (2013). “Sierra Nevada de Santa Marta, Colombia: Conservation Strategy for Tropical Forests,” in Strategies for Sustainability: Latin America (Washington: Routledge), 131–137. Available at: https://www.taylorfrancis.com/chapters/edit/10.4324/9781315066134-18/sierra-nevada-de-santa-marta-colombia-juan-mayr-guillermo-rodriguez-natalia-ortiz-hernando-sanchez.
Mittermeier, R. A., Myers, N., Mittermeier, C. G., and Robles Gil, P. (1999). Hotspots: Earth’s Biologically Richest and Most Endangered Terrestrial Ecoregions. India: CEMEX, 431. ISBN (Hardback): 9-686-39-758-2.
Mittermeier, R. A., Turner, W. R., Larsen, F. W., Brooks, T. M., and Gascon, C. (2011). “Global Biodiversity Conservation: The Critical Role of Hotspots,” in Biodiversity Hotspots. Editors F. Zachos, and J. Habel (Berlin, Heidelberg: Springer). doi:10.1007/978-3-642-20992-5_1
Mukherjee, P. (2010). Postcolonial Environments: Nature, Culture, and the Contemporary Indian Novel in English. Springer. doi:10.1057/9780230251328
Myers, N., Mittermeier, R., Mittermeier, C., da Fonseca, G., and Kent, J. (2000). Biodiversity Hotspots for Conservation Priorities. Nature 403, 853–858. doi:10.1038/35002501
Negret, P. J., Sonter, L., Watson, J. E., Possingham, H. P., Jones, K. R., Suarez, C., et al. (2019). Emerging Evidence That Armed Conflict and Coca Cultivation Influence Deforestation Patterns. Biol. Conserv. 239, 108176. doi:10.1016/j.biocon.2019.07.021
Noll, L., Zhang, S., Zheng, Q., Hu, Y., Hofhansl, F., and Wanek, W. (2022). Climate and Geology Overwrite Land Use Effects on Soil Organic Nitrogen Cycling on a Continental Scale. Biogeosciences 19 (23), 5419–5433. doi:10.5194/bg-19-5419-2022
Nyssen, J., Poesen, J., and Deckers, J. (2009). Land Degradation and Soil and Water Conservation in Tropical Highlands. Soil Tillage Res. 103 (2), 197–202. doi:10.1016/j.still.2008.08.002
Oviedo, G., Noejovich, F., and Zamudio, T. (2007). “Challenges for the Maintenance of Traditional Biodiversity Knowledge in Latin America,” in Indigenous Knowledge Systems and Sustainable Development: Relevance for Africa. Tribes and Tribals (Bogotá: Kamlla-Raj Enterprises), 1, 225–239. Available at: https://scholar.google.com/scholar?hl=es&as_sdt=2005&sciodt=0%2C5&cites=16219705936810416699&scipsc=&q=Oviedo%2C+G.%2C+Noejovich%2C+F.%2C+and+Zamudio%2C+T.+2007.+Challenges+for+the+maintenance+of+traditional+biodiversity+knowledge+in+Latin+America.+Indigenous+knowledge+systems+and+sustainable+development%3A+Relevance+for+Africa.+Tribes+and+Tribals%2C+1%2C+225-239.&btnG=.
Oyuela-Caycedo, A. (2008). “Late Pre-Hispanic Chiefdoms of Northern Colombia and the Formation of Anthropogenic Landscapes,” in The Handbook of South American Archaeology. Editors H. Silverman, and W. H. Isbell (New York, NY: Springer). doi:10.1007/978-0-387-74907-5_22
Pabón, J. D., and Hurtado, G. (2002). La Variabilidad y el Cambio Climático y su Efecto en los Biomas de Páramo, Memorias del Congreso Mundial de Páramos. Bogotá, 98–103. Available at: http://www.lablaa.org/blaavirtual/geografia/congresoparamo/cambio-climatico.htm.
Parks, K. E., and Mulligan, M. (2010). On the Relationship Between a Resource-Based Measure of Geodiversity and Broad Scale Biodiversity Patterns. Biodivers. Conservation 19 (9), 2751–2766. doi:10.1007/s10531-010-9876-z
Pellitero, R., Manosso, F. C., and Serrano, E. (2016). Mid- and Large-Scale Geodiversity Calculation in Fuentes Carrionas (Nw Spain) and Serra do Cadeado (Paraná, Brazil): Methodology and Application for Land Management. Geogr. Ann. Ser. A Phys. Geogr. 97, 219–235. doi:10.1111/geoa.12057
Pérez, A., and Naranjo, C. (2021). “Valoración de la Geodiversidad en la Cuenca del río Ranchería Mediante Herramientas SIG. Bachelor's Thesis. Medellín: Universidad EAFIT. Available at: https://repository.eafit.edu.co/items/8be15da9-4603-42b3-9670-553f80f75bf0 (Accessed April 15, 2024).
Peterson, S. (2002). People and Ecosystems in Colombia: Casualties of the Drug War. Indep. Rev. 6 (3), 427–440. Available at: http://www.jstor.org/stable/24562351.
Piraquive, A., Kammer, A., Bernet, M., Cramer, T., von Quadt, A., and Gómez, C. (2022). Neoproterozoic to Jurassic Tectono-Metamorphic Events in the Sierra Nevada de Santa Marta Massif, Colombia: Insights From Zircon U-Pb Geochronology and Trace Element Geochemistry. Int. Geol. Rev. 64 (14), 1933–1965. doi:10.1080/00206814.2021.1961317
Piraquive-Bermúdez, A. (2016). Structural Framework, deformation and exhumation of the Santa Marta Schists: Accretion and Deformational History of a Caribbean Terrane at the North of the Sierra Nevada de Santa Marta [Ph.D.]. London: Universidad Nacional de Colombia, 394. Available at: https://repositorio.unal.edu.co/handle/unal/59885 (Accessed November 10, 2023).
Pizarro-Bello, D. (2019). “Caracterización de las Geoformas Actuales y Heredadas del Ambiente Glacial de la Sierra Nevada de Santa Marta. Bachelor’s Thesis. Switzerland: Universidad EAFIT. Available at: http://hdl.handle.net/10784/16121.
Poveda, G., and Pineda, K. (2009). Reassessment of Colombia’s Tropical Glaciers Retreat Rates: Are They Bound to Disappear During the 2010–2020 Decade? Adv. Geosciences 22, 107–116. doi:10.5194/adgeo-22-107-2009
Pyhälä, A. (2020). “Resistance to ‘Development’ Amongst the Kogui of the Sierra Nevada de Santa Marta,” in Indigenous Wellbeing and Enterprise (Bogotá: Routledge), 63–87. Available at: https://www.taylorfrancis.com/chapters/edit/10.4324/9780429329029-4/resistance-development-amongst-kogui-sierra-nevada-de-santa-marta-aili-pyh%C3%A4l%C3%A4 (Accessed September 25, 2023).
Quiroga, D. E., Currie, C. A., and Pearse, J. (2024). Lithosphere Removal in the Sierra Nevada de Santa Marta, Colombia. J. Geophys. Res. Solid Earth 129 (1), e2023JB027646. doi:10.1029/2023JB027646
Reichel-Dolmatoff, G. (1982). Cultural Change and Environmental Awareness: A Case Study of the Sierra Nevada de Santa Marta, Colombia. Mt. Res. Dev. 2, 289–298. doi:10.2307/3673093
Restrepo-Moreno, S. A., Foster, D. A., Bernet, M., Min, K., and Noriega, S. (2019). Morphotectonic and Orogenic Development of the Northern Andes of Colombia: A Low-Temperature Thermochronology Perspective. Medellín: Geology and Tectonics of Northwestern South America: The Pacific-Caribbean-Andean Junction, 749–832.
Rocca, L. H. D., and Zielinski, S. (2022). Community-Based Tourism, Social Capital, and Governance of Post-Conflict Rural Tourism Destinations: The Case of Minca, Sierra Nevada de Santa Marta, Colombia. Tour. Manag. Perspect. 43, 100985. doi:10.1016/j.tmp.2022.100985
Rodríguez, A. C. T., Binda, E., Quintero, J. M. O., Garcia, H., Gómez, B., Soto, C., et al. (2020). Answering the Right Questions. Addressing Biodiversity Conservation in Post-Conflict Colombia. Environ. Sci. Policy 104, 82–87. doi:10.1016/j.envsci.2019.11.012
Rodríguez-Osorio, D. (2014). “La Materialidad Prehispánica: Lo Hegemónico y lo Disidente-Campesinos, Indígenas y Científicos,”. Bogotá: Estudio de caso en la Lengüeta, Sierra Nevada de Santa Marta. Available at: https://repositorio.uniandes.edu.co/server/api/core/bitstreams/d602cd14-4f95-4164-ad38-ee3c4a141554/content.
Ruddiman, W. F. (2013). The Anthropocene. Annu. Rev. Earth Planet. Sci. 41, 45–68. doi:10.1146/annurev-earth-050212-123944
Ruiz-Serna, D. (2017). El territorio Como Víctima. Ontología Política y las Leyes de Víctimas para Comunidades Indígenas y Negras en Colombia. Rev. Colomb. Antropol. 53 (2), 85–113. doi:10.22380/2539472x.118
Saba, D. S. (2001). Afghanistan: Environmental Degradation in a Fragile Ecological Setting. Int. J. Sustain. Dev. World Ecol. 8 (4), 279–289. doi:10.1080/13504500109470086
Sanín, M. J., Cardona, A., Céspedes Arias, L. N., González-Arango, C., Pardo, N., and Cadena, C. D. (2024). Volcanoes, Evolving Landscapes, and Biodiversity in Neotropical Mountains. Front. Biogeogr. 16 (1). doi:10.21425/F5FBG61882
Sarmiento, F. O. (2000). “Restoration of Andean Forests for Conservation and Development,” in Forests in Sustainable Mountain Development: A State of Knowledge Report for 2000 (Wallingford UK: CABI Publishing), 59–70. Task Force on Forests in Sustainable Mountain Development. doi:10.1079/9780851994468.0059
Schindler, S., von Wehrden, H., Poirazidis, K., Hochachka, W. M., Wrbka, T., and Kati, V. (2015). Performance of Methods to Select Landscape Metrics for Modelling Species Richness. Ecol. Model. 295, 107–112. doi:10.1016/j.ecolmodel.2014.05.012
Schwanz, V. B., de Oliveira, V. E. S., Simon, A. L. H., and Trentin, G. (2023). A Dinâmica das Coberturas e usos da Terra e as Ameaças à Geodiversidade No Município de São Lourenço do Sul (Brasil). Physis Terrae-Revista Ibero-Afroamericana Geogr. Física Ambiente 5 (2-3), 305–316. doi:10.21814/physisterrae.5574
Serna, D. J., Tamaris-Turizo, C. E., and Gutiérrez Moreno, L. C. (2015). Spatial and Temporal Distribution of Trichoptera (Insecta) Larvae in the Manzanares River Sierra Nevada of Santa Marta (Colombia). Rev. Biol. Trop. 63 (2), 465–477. doi:10.15517/rbt.v63i2.15604
Slaghekke, A. (2020). La construcción de la Territorialidad en la Sierra Nevada de Santa Marta, Colombia. Una Explicación Desde la Antropología del miedo. Anal. Claves Pensam. Contemp. 26 (1-20). Available at: https://hal.science/hal-03141071/.
Spencer, T., Munera, C., Pizarro, V., Lozano-Rivera, P., Esquivel, C., Cardona, O. D., et al. (2024). Coastal Ecosystems Contribution to Climate Adaptation and Disasters Risk Management in the Tropical Americas. Nature-Based Solutions 5, 100112. doi:10.1016/j.nbsj.2024.100112
Steffen, W., Richardson, K., Rockström, J., Schellnhuber, H. J., Dube, O. P., Dutreuil, S., et al. (2020). The Emergence and Evolution of Earth System Science. Nat. Rev. Earth & Environ. 1 (1), 54–63. doi:10.1038/s43017-020-0100-8
Strahler, A. N. (1957). Quantitative Analysis of Watershed Geomorphology. Eos, Trans. Am. Geophys. Union 38 (6), 913–920. doi:10.1029/TR038i006p00913
Suazo-Ortuño, I., Alvarado-Díaz, J., and Martínez-Ramos, M. (2008). Effects of Conversion of Dry Tropical Forest to Agricultural Mosaic on Herpetofaunal Assemblages. Conserv. Biol. 22 (2), 362–374. doi:10.1111/j.1523-1739.2008.00883.x
Suliman, M. (1999). The Rationality and Irrationality of Violence in Civil War: The Darfur Conflict. Dev. Change 30 (4), 711–734.
Tokatlian, J. (2003). The United States and Illegal Crops in Colombia: The Tragic Mistake of Futile Fumigation. UC Berkeley: Center for Latin American Studies. Available at: https://escholarship.org/uc/item/0ft3k11c.
Tschanz, C. M., Marvin, R. F., Cruz, B., Mehnert, J. H. H., and y Cebula, G. T. (1974). Geologic Evolution of the Sierra Nevada de Santa Marta, Northeastern Colombia. Geol. Soc. Am. Bull. 85 (2), 273–284. doi:10.1130/0016-7606(1974)85<273:GEOTSN>2.0.CO;2
Tsegaye, B. (2019). Effect of Land Use and Land Cover Changes on Soil Erosion in Ethiopia. Int. J. Agric. Sci. Food Technol. 5 (1), 026–034. doi:10.17352/2455-815X.000038
Uribe, E. (2005). Natural Resource Conservation and Management in the Sierra Nevada of Santa Marta: Case Study (No. 3269). Medellín: Universidad de los Andes, Facultad de Economía, CEDE. Available at: https://ideas.repec.org/p/col/000089/003269.html (Accessed September 25, 2023).
Valencia-Jaramillo, M., and Castañeda-Molina, D. (2020). Cuantificación de la Geodiversidad en la Cuenta de los Ríos Badillo, Guatapurí, Manaure y Espíritu Santo; PNNN (Parque Nacional Natural Los Nevados) Sierra Nevada de Santa Marta (SNSM) y Serranía del Perijá (SP). Bachelor’s Thesis. Medellín: Universidad EAFIT. Available at: http://hdl.handle.net/10784/24649 (Accessed May 16, 2024).
Valentin, C. (2021). Desarrollo de una Herramienta de ArcGIS para el Cálculo de la Geodiversidad en Latinoamérica, y su Análisis Estadístico y de Correlación con las Amenazas en un Caso de Estudio (Tesis de Maestría). Ciudad de México: Universidad Nacional Autónoma de México, UNAM.
van Beek, R., Cammeraat, E., Andreu, V., Mickovski, S. B., and Dorren, L. (2008). “Hillslope Processes: Mass Wasting, Slope Stability and Erosion,” in Slope Stability and Erosion Control: Ecotechnological Solutions. Editor J. E. Norriset al. (Dordrecht: Springer). doi:10.1007/978-1-4020-6676-4_3
Verweijen, J., and Marijnen, E. (2016). The Counterinsurgency/conservation Nexus: Guerrilla Livelihoods and the Dynamics of Conflict and Violence in the Virunga National Park, Democratic Republic of the Congo. J. Peasant Stud. 45 (2), 300–320. doi:10.1080/03066150.2016.1203307
Vicepresidencia de la República (2002). Panorama Actual de la Sierra Nevada de Santa Marta en Colombia, Conflicto Armado, Regiones, Derechos Humanos y DIH 1998-2002. Bogotá: Observatorio de los Derechos Humanos en Colombia.
Viloria-de-la-Hoz, J. (2005). Sierra Nevada de Santa Marta: Economía de sus Recursos Naturales. Bogotá: Documentos de Trabajo Sobre Economía Regional y Urbana. 61. Available at: https://repositorio.banrep.gov.co/handle/20.500.12134/3163
Viloria de la Hoz, J. (2006). Subregiones Productivas del Caribe Colombiano. Bogotá, D.C: Colección de Economía Regional. Banco de la República, 252. Available at: https://www.banrep.gov.co/sites/default/files/publicaciones/archivos/lbr_subregiones.pdf.
Werner, C., Schmid, M., Ehlers, T. A., Fuentes-Espoz, J. P., Steinkamp, J., Forrest, M., et al. (2018). Effect of Changing Vegetation and Precipitation on Denudation–Part 1: Predicted Vegetation Composition and Cover over the Last 21 Thousand Years Along the Coastal Cordillera of Chile. Earth Surf. Dyn. 6 (4), 829–858. doi:10.5194/esurf-6-829-2018
Wokittel, R. (1957). Bosquejo Geográfico y Geológico de la Sierra Nevada de Santa Marta y la Sierra Nevada de Perijá. Bol. Geol. 5 (3), 22–39. doi:10.32685/0120-1425/bolgeol5.3.1957.323
Worton, G. (2008). “A Historical Perspective on Local Communities and Geological Conservation,”. Editors C. V. Burek, and C. D. Prosser, 300, 137–146. doi:10.1144/SP300.11The History of Geoconservation, Geological Society, London.
Wynn, T. M., Mostaghimi, S., and Alphin, E. F. (2004). “The Effects of Vegetation on Stream Bank Erosion,” in 2004 ASAE Annual Meeting (American Society of Agricultural and Biological Engineers), 1. Available at: https://elibrary.asabe.org/abstract.asp?aid=16423.
Yu, H., Joshi, P. K., Das, K. K., Chauniyal, D. D., Melick, D. R., Yang, X. U. E. F. E. I., et al. (2007). Land Use/Cover Change and Environmental Vulnerability Analysis in Birahi Ganga Sub-Watershed of the Garhwal Himalaya, India. Trop. Ecol. 48 (2), 241. Available at: https://citeseerx.ist.psu.edu/document?repid=rep1&type=pdf&doi=ae1661353472efab7ccaac870f93d80e435c20a0.
Zakharovskyi, V., Kósik, S., Li, B., and Németh, K. (2023). Geosite Determination Based on Geodiversity Assessment Utilizing the Volcanic History of a Near-Sea-Level Explosive Eruption-Dominated Volcanic Island: Tūhua/Mayor Island, New Zealand. Geol. Soc. Lond. Spec. Publ. 530 (1), 127–140. doi:10.1144/SP530-2022-90
Zakharovskyi, V., Németh, K., Gravis, I., and Twemlow, C. (2024). Geosite Recognition Based on Qualitative-Quantitative Assessment in the Light of Core Geological Features of a Mio-Pliocene Volcanic Arc Setting of the Coromandel Peninsula, New Zealand. Geoheritage 16 (1), 19. doi:10.1007/s12371-024-00933-1
Keywords: remote sensing, deforestation, land use change, environmental degradation, Colombian indigenous peoples, socio-environmental conflict, biodiversity hotspots, landscape metrics
Citation: Zapata-Herrera JF, González-Tejada C, Restrepo-Moreno SA and Marín-Cerón MI (2024) The Territory as a Victim: Geological Assessment to Analyzing the Impact of Colombia’s Armed Conflict on Geo-Bio Megadiversity at the Sierra Nevada de Santa Marta. Earth Sci. Syst. Soc. 4:10112. doi: 10.3389/esss.2024.10112
Received: 31 January 2024; Accepted: 11 October 2024;
Published: 07 November 2024.
Edited by:
Adolfo Quesada-Román, University of Costa Rica, Costa RicaReviewed by:
Karoly Nemeth, Institute of Earth Physics and Space Science (EPSS), HungaryDennis Pérez-Umaña, National Emergency Commission (CNE), Costa Rica
Copyright © 2024 Zapata-Herrera, González-Tejada, Restrepo-Moreno and Marín-Cerón. This is an open-access article distributed under the terms of the Creative Commons Attribution License (CC BY). The use, distribution or reproduction in other forums is permitted, provided the original author(s) and the copyright owner(s) are credited and that the original publication in this journal is cited, in accordance with accepted academic practice. No use, distribution or reproduction is permitted which does not comply with these terms.
*Correspondence: Juan Fernando Zapata-Herrera, anphcGF0NTRAZWFmaXQuZWR1LmNv
†ORCID: Juan Fernando Zapata-Herrera, orcid.org/0009-0008-3030-6082; Catalina González-Tejada, orcid.org/0000-0002-3302-248X; Sergio A. Restrepo-Moreno, orcid.org/0000-0001-9828-6681; María Isabel Marín-Cerón, orcid.org/0000-0001-9814-8414