- 1Scripps Institution of Oceanography, University of California, San Diego, La Jolla, CA, United States
- 2Institute of Earth and Environmental Sciences, University of Freiburg, Freiburg im Breisgau, Germany
- 3Department of Earth Sciences, Natural Resources and Sustainable Development, Uppsala University, Uppsala, Sweden
- 4Instituto de Estudios Ambientales y Recursos Naturales (i-UNAT), University of Las Palmas de Gran Canaria (ULPGC), Las Palmas de Gran Canaria, Spain
- 5Centre of Natural Hazard and Disaster Science (CNDS), Uppsala University, Uppsala, Sweden
- 6Departament de Mineralogia, Petrologia i Geologia Aplicada, University of Barcelona, Barcelona, Spain
Incandescent pyroclasts of more than 64 mm in diameter erupted from active volcanoes are known as bombs and pose a significant hazard to life and infrastructure. Volcanic ballistic projectile hazard assessment normally considers fall as the main transport process, estimating its intensity from bomb location and impact cratering. We describe ballistically ejected bombs observed during the late October 2021 episode of eruption at La Palma (Canary Islands) that additionally travelled downhill by rolling and bouncing on the steep tephra-dominated cone. These bouncing bombs travelled for distances >1 km beyond their initial impact sites, increasing total travel distance by as much as 100%. They left multiple impact craters on their travel path and frequently spalled incandescent fragments on impact with substrate, leading to significant fire hazard for partially buried trees and structures far beyond the range of ballistic transport. We term these phenomena as bouncing spallation bombs. The official exclusion zone encompassed this hazard at La Palma, but elsewhere bouncing spallation bombs ought to be accounted for in risk assessment, necessitating awareness of an increased hazard footprint on steep-sided volcanoes with ballistic activity.
Introduction
The threat to human life from volcanoes is of increasing concern, in large part due to the greater than one billion people now living within 100 km of active volcanoes (e.g., Freire et al., 2019), with over 1000 individuals having been killed in eruptions within the past decade (Brown et al., 2017). “Volcano tourism” has further increased risk associated with active volcanoes (e.g., Erfurt-Cooper et al., 2015) and volcanic hazard has proven especially problematic at locations where ballistic or explosive volcanism has occurred with little to no forecasting, including examples from the 1993 Galeras eruption, Colombia (Baxter and Gresham, 1997), the 2012 Upper Te Maari eruption, New Zealand (Fitzgerald et al., 2014), the 2014 Ontake eruption, Japan (Yamaoka et al., 2016), and at Whakaari, New Zealand in 2019 (Dempsey et al., 2020).
Amongst possible volcanic hazards, eruption of ballistic material from volcanic vents has received increasing attention due to the risk these can pose to life, infrastructure, and economy (e.g., Williams et al., 2017). Pyroclasts or fragments of incandescent lava larger than 64 mm in diameter are known as bombs (Wolff and Sumner, 2000; Cas and Wright, 2012; Fisher and Schmincke, 2012) and, due to their size, mass, temperature, and ballistic trajectories, need to be carefully considered during volcanic hazard assessment (Andronico et al., 2013; Gurioli et al., 2014; Fitzgerald et al., 2017, 2020; Taddeucci et al., 2017; Nurmawati and Konstantinou, 2018). Ballistics are a common cause of fatalities close to the vent in global volcanic eruptions (Brown et al., 2017) and are a critical hazard in volcano tourism management (Erfurt-Cooper et al., 2015). They have therefore been of interest for a range of studies, including mechanisms of magma fragmentation (Lavallée et al., 2015), in-flight dynamics (Gurioli et al., 2014; Taddeucci et al., 2017; Cassidy et al., 2018), formation of impact craters by blocks for estimating ballistic hazard (Fitzgerald et al., 2014), as well as the dangers that they present for the destruction of infrastructure and the threats to local populations, livestock, and wildlife (Vanderkluysen et al., 2012; Williams et al., 2017; Yamada et al., 2018; Fitzgerald et al., 2020; Deligne et al., 2022).
Here we report on bomb phenomena observed during the 2021 eruption of La Palma (Canary Islands, Spain) that has not been previously described. Descriptive documentation is provided of bombs that were observed rolling and bouncing down the tephra-covered slopes of the newly formed Tajogaite volcanic cone after initial ballistic transport and first contact with the ground, and which were seen spalling incandescent material during their journey downhill. We term these volcanic ejecta “bouncing spallation bombs,” defined as incandescent magmatic fragments >64 mm in diameter ejected in explosive eruptions that travel initially ballistically, followed by downslope travel through bouncing and rolling during which occasional spallation of incandescent material occurs. Due to the bouncing and associated deflection on uneven ground, these bouncing spallation bombs have relatively unpredictable trajectories and their final travel distance is usually considerably greater than what would be assumed from ballistic transport of volcanic projectiles alone. Raising awareness of this type of bomb phenomena is important as it has been only rarely observed and described at other volcanoes (e.g., Francis, 1973; Fries et al., 1993). Our observations provide additional insight into the formation, transport and hazard footprint of this specific form of volcanic ejecta. The observation of these bombs also has implications for understanding the role of tephra substrate on bomb transport and on the use of impact crater counting to estimate the number of ballistic projectiles produced during eruptions.
Background and Methods
Background of the 2021 La Palma Eruption and Elemental Abundances
The 2021 eruption on La Palma began at ∼14:11 UTC on the 19th September and ended at ∼22:21 UTC on 13th December 2021 (Longpre, 2021; Romero et al., 2022). It is the largest, longest, and most destructive eruption on the island in recorded history, ahead of previous historical eruptions in AD 1585, 1646, 1677-1678, 1712, 1949, and 1971, all of them emanating from the active Cumbre Vieja volcanic rift system (Carracedo et al., 2001). The eruption of extensive lava flows and thick layers of ash and lapilli led to significant destruction, with the loss of ∼3000 buildings, >70 km of roads, and ∼12 km2 of crops (1237 Ha) (e.g., Carracedo et al., 2022). Compositional data on the bomb material and associated underlying lapilli and tephra are provided in the Supplementary Material, including details of the analytical methods and comparison with a more comprehensive dataset of lava compositions from the entirety of the 2021 La Palma eruption (Day et al., 2022).
Observations and Video Preparation
Direct observations during the 2021 La Palma eruption were made during the 26th to the 28th of October 2021 in daylight and were recorded through photographs and videos. All media was geotagged and edited to include observation date and time and converted to common digital formats to ensure playback on most devices. Videos are provided as links, due to their size, in the Supplementary Material. Due to the hazards surrounding the falling bombs, some quantitative aspects (mass, size and shape of bombs) could not be accurately documented at the time of observation for safety reasons. Estimation of velocity and distance was based on both field observations and video analysis, either by directly pacing tracks, or measuring the location and distances of features and the time taken to travel between these known locations.
Observations and Results
Direct observations of bouncing spallation bombs were made in the official safety exclusion zone to the northeast of the village of Las Manchas on the western flank of the Cumbre Vieja on La Palma (28°36′32.8″N, 17°52′27.6″W), approximately 900 m southwest of the new basaltic cinder cone (28°36′54″N, 17°52′7″W) (Figure 1). Visits were made before (26th October), during (27th October) and after the fall of the volcanic bombs at the site (28th October). The observation of bouncing spallation bombs occurred between 14:30 to 15:00 local time on 27th of October 2021 and took place during a period of fire fountaining when a new lava flow was emanating from the north-western flank of the cinder cone and flowed through the northern outskirts of the village of Las Manchas [see Carracedo et al. (2022) for details of the overall eruption]. On 26th October, part of the author team (JD, HG, FJPT, MA, and GG) had visited the same area and found it to be subject to intense (>4 cm/day) basaltic lapilli fall compared to previous and subsequent days. During the afternoon of 27th October, smaller ash-sized basaltic particles were falling from the eruption plume (Supplementary Figure S1).

FIGURE 1. Map of the Canary Islands (A) showing the location of the 2021 eruption on La Palma Island (B). Key features of the whole volcanic field: the extent of the lava field on 27th October 2021 (blue stippled line), the official near vent safety exclusion zone (red stippled line), and the region of bouncing spallation bomb observations (red box) are shown in panel (B).
At approximately 14:30, 27th October, another part of the team (JD, HG, VT, and JCC) approached the flank of the volcano following the route of the LP-212 road. It was noted that ∼20 roughly spherical 30–70 cm diameter bombs, still warm to the touch, had been freshly deposited, as they were not present the previous day. They were resting on top of tephra that was observed to have fallen the previous day. These bombs were well-consolidated basaltic rocks and were distributed across a region where the steep-sided cinder cone graded into flatter ground. The field party noted ejection of large ballistics (>1 m across) from the main active vent (located approximately 1 km up slope from the observation area), which fell onto the flanks of the steepening cinder cone, within about 200–500 m of the vent (Figure 2). It was also noted that elliptical down-slope indents or hollows occurred in the basaltic lapilli and coarse ash substrate in the area where the team was observing.

FIGURE 2. The eruption column and associated pyroclastic fall on 27th October 2021, with large bombs in the foreground. The track of the rolling bomb in (A) is partially visible on the right-hand side of the bomb. The track of the bomb in (B) is visible running downslope. Unlike conditions on the previous day, where ejected material occurred all along the NW-SE trending crater complex, during the 27th October material was dominantly ejected from the center and NW end of the crater system (also see Supplementary Video Content).
The presence of the indent trails consistently upslope of the warm bombs at the observation location, and the ballistic activity at the volcanic vent, suggested that indents had been formed by bombs bouncing downslope. Some bombs showed no obvious hollows behind them, suggesting that they had fallen significantly prior to the team’s arrival in the area, with hollowed bounce marks having already been filled by the steadily falling ash and lapilli. Observed indent trails showed that some of the volcanic bombs had travelled substantially more than 10 m per bounce in the area of observation, as determined by pacing out the path length and later confirmed by estimation from video imagery (Supplementary Video S1). The exact bombs responsible for some of the tracks could not be identified in all cases, as they had rolled and bounced further downslope. The length of bouncing tracks and absence of the bouncing spallation bombs responsible for them, beyond a line-of-sight at least 0.5 km downslope of the main observation area, indicated that they had travelled total distances of more than 1.5 km from the vent site (Supplementary Video S1).
At 14:34 the team noted a large bomb that was ejected and tumbled down the southwestern flank of the cinder cone (Supplementary Video S2). Ejection occurred from the central vent, which, at the time, was producing ash-laden eruption jets. After the initial fall and contact with the ground, the bomb produced a plume of dust that was left behind during the tumbling and bouncing phases. After this bomb came to rest, it was approached. The bomb formed a progressively more closely spaced “rolling” track as it slowed down and eventually halted near the field party’s position. Indents were initially spaced meters apart and got closer together as the bomb slowed and finally came to rest (e.g., Figure 2B). The bomb was ∼60 cm in diameter, with an aphanitic, shiny and dark scoriaceous outer crust and a lighter grey outer basaltic shell, but with incandescent basaltic material in the center (Figure 3A). There was no indication for any significant accretion to the bomb. The bomb was imaged and carefully hammered to remove a sample that was quenched by pouring water on it (LP2106JD; Figure 3B).
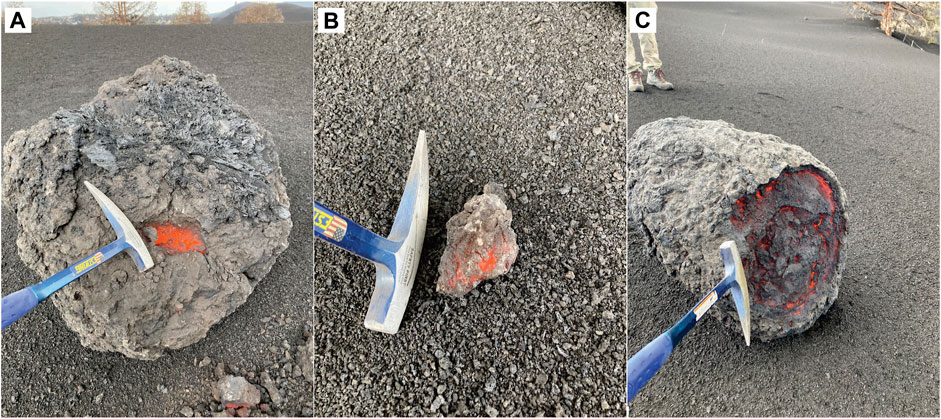
FIGURE 3. Spallation bombs examined on the 27th October 2021 immediately after coming to rest. (A) Spallation bomb that was observed to have travelled downhill at 14:34 local time, showing an incandescent inner core with a dark and shiny scoriacous coating. (B) Sample from bomb shown in (A). (C) Spallation bomb observed to fall at 14:56 local time and that rested approximately 70 m NW of (A). Note the incandescent center and the apparent concentric interior pattern. The broken off outer crust is an indication for spallation during transport and could be a feature that can help recognition of this phenomenon in volcanic terrains elsewhere. Hammer head is 19 cm in length.
During observation of the bomb that fell at 14:34, other bombs travelling downhill in a similar bouncing fashion were seen at 14:42 (Supplementary Video S2), and at 14:54 local time (Supplementary Video S3). The 14:54 bomb formed a dust trail during its transit down the slope of the cone and was seen to bounce and to spall incandescent material. Based on estimation of travel velocity from video analysis, the bomb travelled initially at speeds of more than 60 km/h prior to decelerating on the shallowing slope and finally coming to rest on the soft lapilli-sized particle substrate. This bomb halted within a stand of dead trees and combustion of woody material took place as later evidenced by smoke emerging from the site (Supplementary Video S3). Two minutes later (14:56 local time; Supplementary Video S4) another bomb was observed to follow a similar track and speed down the side of the cone. Like the previous bomb, the object spalled incandescent material and was slowed down by the substrate as well as by collision with a pre-existing lava flow (Figures 4A–D). On halting, the bomb was approached and found to be ovoid in shape, around 70 cm in length and 40 cm in width. One side of the bomb, which had been observed to spall during transit and impact with the ground and the lava flow, exposed an incandescent interior (Figure 3C).

FIGURE 4. Image stills from videos showing (A) downward movement of the bomb, (B) episodic spalling while bouncing over soft tephra substrate, (C) further downhill movement and (D) leaving behind the incandescent fragment (shrapnel) that spalled off the bomb while the main bomb approached the tephra-covered lava flow (also see Supplementary Video). The red stippled circle highlights and tracks the spalled shrapnel fragment.
Returning to the site at 14:00 on 28th October 2021 revealed that minor ashfall had occurred during the preceding ∼23 h period. No more than about half-a-dozen new bouncing spallation bombs were noted in the area, suggesting that the main phase of activity to produce the bouncing spallation bombs was between the evening of 26th October 2021 and ∼15:00 on 27th October 2021, a period of <24 h. The ashfall was sufficiently intense to fill most of the hollows observed to have formed in the previous day, emphasising the perishable nature of this form of information. For chemical analysis of a representative bomb sample and the underlying tephra, see Supplementary Material to this article.
Discussion
The basaltic bombs that fell on the 27th October 2021 at La Palma were observed to travel ballistically, and then to bounce and roll as solid incandescent materials (Figure 5) while being cushioned, in part, by the relatively soft lapilli substrate. They were bombs rather than accretionary balls as they lost material during final transport (the term “egg” has been used in the popular press but does not capture the diverse shapes of objects observed). They are therefore distinct in their mode of transport from accretionary lava balls such as “Teide’s Eggs” or lava bombs observed during the 1971 eruption on La Palma (Carracedo and Troll, 2016; Carracedo et al., 2019). Bouncing spallation bombs travel freely downslope by bouncing and rolling after ballistic ejection and become disassociated from other vent products. We therefore suggest the term ‘bouncing spallation bomb’ for this bouncing, rolling and spalling ejecta (see Introduction for definition). This term is consistent with the need for relatively viscous lava to provide shape (opposite to solid material in a ballistic block) and with the observation of fragmentation or spalling during rolling and bouncing (Supplementary Videos S2, S4).

FIGURE 5. Representation of spallation bomb travel paths during the 27th October 2021 eruption on La Palma and the extended hazard posed by them. Orange lines denote ballistic fall trajectories, the red stippled line denotes the rolling and bouncing phase of transport. Black stippled arrows link the schematic representations to actual observation of phenomena in the photographic image. The spallation range can extend from ∼200 m from the vent to more than 1500 m, judging from our observations at La Palma in October 2021.
Bouncing spallation bombs during the 27th October 2021 were mostly preserved due to their transport and deposition onto a soft substrate of ash and lapilli. Tephra cushioning during ballistic impacts has been considered in the context of acting to protect structures (Williams et al., 2019), but our observations show it may also be important in facilitating bouncing, rolling, and spalling of bombs. In combination with steeper slope angles, soft tephra substrates are likely to facilitate a larger transport distance and hazard footprint. In addition, the characteristics of bouncing spallation bombs (see Supplementary Videos) suggests that they do not spall solely through centrifugal forces, but also on impact with the ground as seen in the imagery (e.g., Figure 4). This means that their preservation potential would strongly diminish if impacting on harder material such as a solid lava flow, as they would likely shatter and produce a shrapnel effect, which in turn would probably result in shorter travelled distances.
An important aspect of the bouncing spallation bombs is the presence of a “track” formed during tumbling and rolling down the slope of the cone. These tracks are formed by indents within ash and lapilli deposits. Preservation of such depressions in deposits can be useful for understanding the potential for spallation bomb activity during future volcanic eruptions (e.g., Fitzgerald et al., 2014), even on Mars, where depressions have been interpreted as bomb sag at Home Plate (Manga et al., 2012). The potential of bombs to bounce and roll downslope, creating multiple impacts (or a track) should be considered when using bomb sags to assess ballistic hazard. For example, Fitzgerald et al. (2014) used field observations at Tongariro volcano, New Zealand, to show that more than four times as many impact craters were detected during field inspection than from orthophoto mapping of the same region. These authors estimated the number of projectiles that affected a certain area based on the number of craters considering that each impact corresponded to a separate volcanic block. During the 2021 La Palma eruption, as well as in locations where historic ballistic projectiles have been mapped (e.g., Galindo et al., 2013), however, the action of rolling and bouncing may have created numerous craters from a single projectile, implying the number of impact sites cannot always be directly correlated with the number of erupted projectiles, leading to a distorted assessment of associated hazard. Taking multiple impacts from bouncing spallation bombs into consideration, in addition to crater and track preservation issues from subsequent tephra deposition, is likely to be more realistic in future studies that use impact craters as a measure of bomb activity.
Direct observations of emission of gravitationally-induced rolling hazards have been made previously in the Canary Islands. For instance, during the 1971 eruption of Teneguía volcano in La Palma, when large (>1 m diameter) incandescent lava blocks were seen to roll down the western slope of the cone by one of the authors (JCC) and captured in video (Supplementary Video S5). In detail, these hazards from the Teneguía eruption differ from the bouncing spallation bombs described here in their origin by detachment from a lava flow that traveled downslope as well as their shape, size, and the shorter distance that they travelled, but have commonality in rolling downslope and in that they can spall incandescent material. Additionally, reports from the 5th May to 13th June 1706 Garachico eruption on Tenerife mention “lava balls” several meters in diameter that rolled down the slope and spalled red-hot fragments that produced fires in the village before the main lava flows reached the coast (Cassares, 1709), appear similar to the 1971 Teneguía eruptive products, but are distinct from the bouncing spallation bombs described here.
The bouncing spallation bombs described from La Palma also show similarities to examples from locations other than the Canary Islands. Examples include Pacaya, Guatemala, where they were termed “Cannonball” bombs (Francis, 1973), as well as from Paracutin, Mexico (Fries et al., 1993), La Réunion, Indian Ocean (Edwards et al., 2020), on Stromboli, Italy (Vanderkluysen et al., 2012), and Bogoslof, Alaska (Waythomas and Mastin, 2020). However, evidence for extended travel of the bombs after ballistic flight, and associated spallation, has not been widely constrained. This is problematic when considering ballistic transport from vents for older eruptions where the bomb transport was not observed, as it could lead to erroneous assumptions about ballistic trajectories and the extent of true hazard. Without recognition of the potential continued transport (rolling and bouncing) after impact, ballistic trajectory distances may be overestimated, and hazard from bouncing spallation bombs underestimated or not considered at all.
As an example of the difficulty in recognizing bouncing spallation bomb phenomena in the geological record, a notable feature of bouncing spallation bombs at La Palma is that they came to rest proximal to the edge of a pre-existing lava flow. Had the fall of these bouncing spallation bombs not been witnessed directly, then their concentric inner portions and proximity to the lava flow might well have led to their misidentification as accretionary bombs or “lava balls” associated with the lava flow, making it important to be aware of the phenomenon despite the rare number of direct observations currently available. Hazard assessment related to volcanic bombs typically considers ballistic transport from the source vent (e.g., Fitzgerald et al., 2014, 2020; Gurioli et al., 2014; Taddeucci et al., 2017; Williams et al., 2019), yet during the event of the 27th of October 2021, the field party noted several potentially significant and hitherto underappreciated characteristics surrounding the formation of bouncing spallation bombs. In the first instance, their ballistic ejection was followed by gravitational tumbling, rolling and bouncing on the soft tephra substrate, which meant they had the potential to travel far greater distances than ballistically transport, especially on the steep flanked cone of the 2021 eruption that itself took place on the flank of the Cumbre Vieja ridge. As such, they represent a significant hazard well beyond the area of ballistic transport. In addition to expecting projectiles to fall from above, bouncing spallation bombs are also hazards that travel parallel to the ground. Ballistic hazard assessment should therefore take into consideration this additional gravitational impetus and the different directional threat during relatively explosive basaltic eruptions.
In the case of the 2021 La Palma eruption, the near vent exclusion zone within which bouncing spallation bombs were observed was limited to civil protection personnel and permitted scientists studying the volcanic phenomena. This exclusion zone was large enough to accommodate the bouncing spallation bomb hazard due to the extent of downslope lava flows, and is likely to be a useful consideration in future volcanic risk management. In the case of the observations made here, team members were aware of the hazard and worked only during a daylight period with good visibility when they were able to spot the bouncing spallation bombs. Elsewhere, globally, where this might not be the case, onset of spallation bomb activity is conceivable during night-time eruption phases and, as the videos in the Supplementary Material demonstrate, their exact travel trajectory and that of spalling projectiles is unpredictable. This presents a challenge in eruptions without careful monitoring, especially with volcano tourism on the rise, bringing more people to more remote volcanoes than ever before. In appropriate cases, such as future Canary Island eruptions, a useful risk mitigation measure would be full time visible and infrared video surveillance, and the widening of volcanic exclusion zones beyond the areas affected solely by ballistically transported projectiles. In addition, the fragmentation and spallation of the bombs can also lead to the potential for multiple spalled hot fragments and shrapnel outside of the immediate (ballistic) danger zone, adding a “hazard envelope” related to the trajectory of individual bouncing spallation bombs.
A final critical aspect of hazard is the hot, incandescent nature of the bombs. One bomb was witnessed to land in a dead tree stand (Supplementary Video S3), which was later observed by several members of the field party to ignite the woody materials that were close to it. The potential to ignite forests, timber structures or other flammable constructions would be severe from spallation bombs, since such objects travel further than other bomb types and, due to spallation and fragmentation, can lead to incandescent material spalling and igniting fires at several sites simultaneously (cf. Giordano and De Astis, 2021). This issue is exemplified well beyond La Palma. At Mt. Ontake, Japan, casualties have been recorded from falling blocks, and consequently, improved designs have been proposed for wooden structures to protect from ballistic hazards (Yamada et al., 2018). In addition to these improvements, the hazard posed by ‘high temperature ballistics’ could also be considered in future building requirements.
Conclusions
Bouncing spallation bombs of the 2021 La Palma eruption show that the hazard radius from volcanic ballistics significantly increases—by as much as a factor of two—from what is normally assumed for ballistic trajectories alone. Spallation bomb events such as those witnessed in October 2021 should be considered in hazard risk assessment at active volcanoes of similar type to La Palma, as well as in the study of historical volcanic bomb deposits. The less familiar risk of the bouncing spallation bomb hazard is particularly important where volcanoes and society (including volcano tourism) intersect. Quantitative analysis of bouncing spallation bomb transport in future eruptions will be useful to further assess this phenomenon. At La Palma, the authorities took a sensible approach to volcanic hazard, enabling observations of the bouncing spallation bomb phenomena while simultaneously keeping people safe throughout the entire eruption. We recommend that such an approach is also considered for comparable volcanic situations elsewhere.
Data Availability Statement
The original contributions presented in the study are included in the article/Supplementary Material, further inquiries can be directed to the corresponding author.
Author Contributions
Field work was carried out by all authors. JD performed geochemical analyses and wrote the manuscript draft. HG performed video and photo editing. All authors participated in the interpretation of the data and provided input on the manuscript.
Funding
Field observations were supported by NSF EAR 1918322 (JD), Friedrich-Rinne-Stiftung at the University of Freiburg (HG), Vetenskapsrådet Sweden and Uppsala University (VT), the LAJIAL PGC 2018-101027-B-I00, MCIU/AEI/FEDER, EU (FP-T and MA) and the MESVOL-ULPGC (FP-T) research projects.
Conflict of Interest
The authors declare that the research was conducted in the absence of any commercial or financial relationships that could be construed as a potential conflict of interest.
Publisher’s Note
All claims expressed in this article are solely those of the authors and do not necessarily represent those of their affiliated organizations, or those of the publisher, the editors and the reviewers. Any product that may be evaluated in this article, or claim that may be made by its manufacturer, is not guaranteed or endorsed by the publisher.
Acknowledgments
We are grateful to Ben Kennedy, Ulrich Küppers, Rebecca Fitzgerald, and George Williams for constructive comments on versions of this manuscript. Discussions with Vicente Soler, Claus Siebe, Frances Deegan, and Mike Heap are gratefully acknowledged. The authors thank PEVOLCA for enabling access to the Exclusion Zone of La Palma during the 2021 eruption for continuous sample collection.
Supplementary Material
The Supplementary Material for this article can be found online at: https://www.escubed.org/articles/10.3389/esss.2022.10063/full#supplementary-material
References
Andronico, D., Scollo, S., and Cristaldi, A. (2015). Unexpected Hazards from Tephra Fallouts at Mt Etna: The 23 November 2013 Lava Fountain. J. Volcanol. Geotherm. Res. 304, 118–125. doi:10.1016/j.jvolgeores.2015.08.007
Baxter, P. J., and Gresham, A. (1997). Deaths and Injuries in the Eruption of Galeras Volcano, Colombia, 14 January 1993. J. Volcanol. Geotherm. Res. 77 (96), 325–338. doi:10.1016/S0377-0273(96)00103-5
Brown, S. K., Jenkins, S. F., Sparks, R. S. J., Odbert, H., and Auker, M. R. (2017). Volcanic Fatalities Database: Analysis of Volcanic Threat with Distance and Victim Classification. J. Appl. Volcanol. 6, 15. doi:10.1186/s13617-017-0067-4
Carracedo, J. C., Rodriguez-Badiola, E., Guillou, H., Nuez Pestana, J. D. L., and Pérez Torrado, F. J. (2001). Geology and Volcanology of La Palma and El Hierro, Western Canaries. Estud. Geol. 57, 175.
Carracedo, J. C., Troll, V. R., Day, J. M. D., Geiger, H., Aulinas Junca, M., Soler, V., et al. (2022). The 2021 Eruption of the Cumbre Vieja Volcanic Ridge on La Palma, Canary Islands. Geol. Today 38, 94–108. doi:10.31223/X5D06D
Carracedo, J. C., Troll, V. R., and Socorro, S. (2019). Geosites of Teide Volcano and Tenerife. Ediciones Saquiro, 265.
Carracedo, J. C., and Troll, V. R. (2016). The Geology of the Canary Islands. Elsevier, 621pp. doi:10.1016/C2015-0-04268-X
Cas, R., and Wright, J. (2012). Volcanic Successions Modern and Ancient: A Geological Approach to Processes, Products and Successions. Springer Science & Business Media.
Cassares, D. J. (1709). Breve narrativa de la lamentable y lastimosa desgracia de Garachico. Biblioteca de la Universidad de La Laguna. Ms 83.II.10 (F.73-87r).
Cassidy, M., Manga, M., Cashman, K., and Bachmann, O. (2018). Controls on Explosive-Effusive Volcanic Eruption Styles. Nat. Commun. 9, 2839–2916. doi:10.1038/s41467-018-05293-3
Day, J. M. D., Troll, V. R., Aulinas, M., Deegan, F., Geiger, H., Carracedo, J. C., et al. (2022). Mantle Source Characteristics and Magmatic Processes during the 2021 La Palma Eruption. Earth Planet. Sci. Lett. 597, 117793. doi:10.1016/j.epsl.2022.117793
Deligne, N. I., Jenkins, S. F., Meredith, E. S., Williams, G. T., Leonard, G. S., Stewart, C., et al. (2022). From Anecdotes to Quantification: Advances in Characterizing Volcanic Eruption Impacts on the Built Environment. Bull. Volcanol. 84, 7–9. doi:10.1007/s00445-021-01506-8
Dempsey, D. E., Cronin, S. J., Mei, S., and Kempa-Liehr, A. W. (2020). Automatic Precursor Recognition and Real-Time Forecasting of Sudden Explosive Volcanic Eruptions at Whakaari, New Zealand. Nat. Commun. 11, 3562–3568. doi:10.1038/s41467-020-17375-2
Edwards, M. J., Pioli, L., Harris, A. J. L., Gurioli, L., and Thivet, S. (2020). Magma fragmentation and particle size distributions in low intensity mafic explosions: the July/August 2015 Piton de la Fournaise eruption. Sci. Rep. 10, 13953. doi:10.1038/s41598-020-69976-y
Erfurt-Cooper, P., Sigurdsson, H., and Lopes, R. M. (2015). Volcanoes and Tourism.” in The Encyclopedia of Volcanoes. Elsevier, 1295.
Fitzgerald, R. H., Kennedy, B. M., Gomez, C., Wilson, T. M., Simons, B., Leonard, G. S., et al. (2020). Volcanic Ballistic Projectile Deposition from a Continuously Erupting Volcano: Yasur Volcano, Vanuatu. Volcanica 3, 183–204. doi:10.30909/vol.03.02.183204
Fitzgerald, R. H., Kennedy, B. M., Wilson, T. M., Leonard, G. S., Tsunematsu, K., and Keys, H. (2017). “The Communication and Risk Management of Volcanic Ballistic Hazards,” in Observing the Volcano World (Cham: Springer), 121–147.
Fitzgerald, R. H., Tsunematsu, K., Kennedy, B. M., Breard, E. C. P., Lube, G., Wilson, T. M., et al. (2014). The Application of a Calibrated 3D Ballistic Trajectory Model to Ballistic Hazard Assessments at Upper Te Maari, Tongariro. J. Volcanol. Geotherm. Res. 286, 248–262. doi:10.1016/j.jvolgeores.2014.04.006
Francis, P. W. (1973). Cannonball Bombs, a New Kind of Volcanic Bomb from the Pacaya Volcano, Guatemala. Geol. Soc. Am. Bull. 84, 2791–2794. doi:10.1130/0016-7606(1973)84<2791:cbanko>2.0.co;2
Freire, S., Florczyk, A. J., Pesaresi, M., and Sliuzas, R. (2019). An Improved Global Analysis of Population Distribution in Proximity to Active Volcanoes, 1975–2015. ISPRS Int. J. Geoinf. 8 (8), 341. doi:10.3390/ijgi8080341
Fries, C., Segerstrom, K., Tilling, R. I., and Wilcox, R. E. (1993). Movie Footage of the Activity of Paricutin Volcano, Michoacan, Mexico, 1945-1952. US Department of the Interior, US Geological Survey.
Galindo, I., Romero, M. C., Sánchez, N., Dóniz, J., Yepes, J., Morales, J. M., et al. (2013). Morphology and distribution of volcanic bombs in Caldera Quemada de Arriba (Lanzarote, Canary Islands): implications for volcanic hazard analysis. Environmental security, geological hazards and management, 207.
Giordano, G., and De Astis, G. (2021). The Summer 2019 Basaltic Vulcanian Eruptions (Paroxysms) of Stromboli. Bull. Volcanol. 83, 1–27. doi:10.1007/s00445-020-01423-2
Gurioli, L., Colo', L., Bollasina, A. J., Harris, A. J. L., Whittington, A., and Ripepe, M. (2014). Dynamics of Strombolian Explosions: Inferences from Field and Laboratory Studies of Erupted Bombs from Stromboli Volcano. J. Geophys. Res. Solid Earth 119, 319–345. doi:10.1002/2013jb010355
Lavallee, Y., Dingwell, D. B., Johnson, J. B., Cimarelli, C., Hornby, A. J., Kendrick, J. E., et al. (2015). Thermal Vesiculation during Volcanic Eruptions. Nature 528, 544–547. doi:10.1038/nature16153
Longpre, M.-A. (2021). Reactivation of Cumbre Vieja Volcano. Science 374, 1197–1198. doi:10.1126/science.abm9423
Manga, M., Patel, A., Dufek, J., and Kite, E. S. (2012). Wet Surface and Dense Atmosphere on Early Mars Suggested by the Bomb Sag at Home Plate, Mars. Geophys. Res. Lett. 39 (1). doi:10.1029/2011GL050192
Nurmawati, A., and Konstantinou, K. I. (2018). Hazard Assessment of Volcanic Ballistic Impacts at Mt Chihshin, Tatun Volcano Group, Northern Taiwan. Nat. Hazards (Dordr). 92, 77–92. doi:10.1007/s11069-018-3192-4
Romero, J. E., Burton, M., Cáceres, F., Taddeucci, J., Civico, R., Ricci, T., et al. (2022). The Initial Phase of the 2021 Cumbre Vieja Ridge Eruption (Canary Islands): Products and Dynamics Controlling Edifice Growth and Collapse. J. Volcanol. Geotherm. Res. 431, 107642. doi:10.1016/j.jvolgeores.2022.107642
Taddeucci, J., Alatorre-Ibargüengoitia, M. A., Cruz-Vázquez, O., Del Bello, E., Scarlato, P., and Ricci, T. (2017). In-flight Dynamics of Volcanic Ballistic Projectiles. Rev. Geophys. 55, 675–718. doi:10.1002/2017RG000564
Vanderkluysen, L., Harris, A. J. L., Kelfoun, K., Bonadonna, C., and Ripepe, M. (2012). Bombs Behaving Badly: Unexpected Trajectories and Cooling of Volcanic Projectiles. Bull. Volcanol. 74, 1849–1858. doi:10.1007/s00445-012-0635-8
Waythomas, C. F., and Mastin, L. G. (2020). Mechanisms for Ballistic Block Ejection during the 2016–2017 Shallow Submarine Eruption of Bogoslof Volcano, Alaska. Bull. Volcanol. 82, 13–20. doi:10.1007/s00445-019-1351-4
Williams, G. T., Kennedy, B. M., Lallemant, D., Wilson, T. M., Allen, N., Scott, A., et al. (2019). Tephra Cushioning of Ballistic Impacts: Quantifying Building Vulnerability through Pneumatic Cannon Experiments and Multiple Fragility Curve Fitting Approaches. J. Volcanol. Geotherm. Res. 388, 106711. doi:10.1016/j.jvolgeores.2019.106711
Williams, G. T., Kennedy, B. M., Wilson, T. M., Fitzgerald, R. H., Tsunematsu, K., and Teissier, A. (2017). Buildings vs. Ballistics: Quantifying the Vulnerability of Buildings to Volcanic Ballistic Impacts Using Field Studies and Pneumatic Cannon Experiments. J. Volcanol. Geotherm. Res. 343, 171–180. doi:10.1016/j.jvolgeores.2017.06.026
Wolff, J. A., and Sumner, J. M. (2000). “Lava Fountains and Their Products,” in Encyclopaedia of Volcanoes (Academic Press), 321.
Yamada, H., Tateyama, K., Sasaki, H., Naruke, S., Kishimoto, H., and Yoshimoto, M. (2018). Impact Resistance to Ballistic Ejecta of Wooden Buildings and a Simple Reinforcement Method Using Aramid Fabric. J. Volcanol. Geotherm. Res. 359, 37–46. doi:10.1016/j.jvolgeores.2018.06.014
Keywords: volcanic, bomb, hazard, La Palma, Canary Islands
Citation: Day JMD, Geiger H, Troll VR, Perez-Torrado FJ, Aulinas M, Gisbert G and Carracedo JC (2022) Bouncing Spallation Bombs During the 2021 La Palma Eruption, Canary Islands, Spain. Earth Sci. Syst. Soc. 2:10063. doi: 10.3389/esss.2022.10063
Received: 03 July 2022; Accepted: 24 November 2022;
Published: 08 December 2022.
Edited by:
Natasha Dowey, Sheffield Hallam University, United KingdomReviewed by:
Rebecca Fitzgerald, GNS Science, New ZealandGeorge Williams, Verisk Analytics, United States
Copyright © 2022 Day, Geiger, Troll, Perez-Torrado, Aulinas, Gisbert and Carracedo. This is an open-access article distributed under the terms of the Creative Commons Attribution License (CC BY). The use, distribution or reproduction in other forums is permitted, provided the original author(s) and the copyright owner(s) are credited and that the original publication in this journal is cited, in accordance with accepted academic practice. No use, distribution or reproduction is permitted which does not comply with these terms.
*Correspondence: James M. D. Day, am1kZGF5QHVjc2QuZWR1