- School of Natural and Built Environment, Queen’s University, Belfast, United Kingdom
Inadequate base maps with poor scale or low resolution demonstrate the need for contemporary topographic maps when conducting geological mapping. In neotectonic regimes and areas of dynamic geomorphology, archival or large-scale maps require time-consuming, on-site manual updating while mapping bedrock and superficial geology. In contrast, stable ground conditions may have suitable legacy maps in some locations but not in others, such as where surveying is absent, incomplete or subject to legal restrictions. The geologist tasked with mapping may have to do this on short notice at their first site visit with no time to search for or create digital or physical copies of background maps on a suitable scale. The field mapper may encounter any of the above scenarios, especially the Geoforensic specialist tasked with Search and Rescue, hazard assessment or preparation and desktop study for subsequent search teams or law enforcement. Drone-derived orthoimagery and digital surface modelling can be conducted on-site in near real time to provide high resolution georeferenced maps for direct input of geological information, thus bypassing either non-existent or unsuitable base maps.
Background
The field geologist or geomorphologist may often find themselves in a location where base maps for the recording of spatial data are inadequate. A potential solution to such scenarios was outlined by Jones et al. (2004), whose workflow model is not dissimilar to that developed here, where external digital data sources such as drone orthoimagery or satellite imagery are uploaded to a GNSS-enabled field device (such as a tablet) or where external GNSS is used to spatially record field data. A similar approach was taken by Whitmeyer et al. (2010), who focused on their sophisticated method of building geologic models in Google Earth using field-recorded digital data, while Nesbit et al. (2018) combined spatial and stratigraphic information in their study at Dinosaur Provincial Park (Alberta, Canada) in a similar approach to ours. Bemis et al. (2014) and Crillio et al. (2022) used structure-from-motion software to combine ground-based georeferenced digital photography with drone aerial surveys in their analysis of structural geology, thus integrating datasets at varying scales, which is pertinent to this work (see Conclusions and Recommendations). In mapping for Forensic Geology (or Geoforensics, see definitions below) these approaches are applicable, and we have outlined case studies and a generic workflow highlighting the importance of having updated digital base maps in the field on which real-time observations can be made. Methods similar to this work have been used in mineral exploration (Sikakwe, 2023) and the overall approach is well-established in geotechnical and civil engineering, where published maps require continuous updating during construction and commonly at an inappropriate scale (Dearman, 1991). Our work extends the above published studies by using an iterative on-site approach, where the forensic geologist may be conducting a preliminary walk-over survey, followed immediately by geological mapping as part of Search and Rescue and/or crime scene mapping. This is the first use of such an approach in forensic geology.
Forensic Geology and Geoforensics
Forensic Science is the combined application of scientific techniques to support legal processes (Houck and Siegel, 2010), or the systematic collection of data and formulation of testable theories to understand the physical world, here “as applied to public or legal concerns” (Houck and Siegel, 2010 p.4). Forensic Geology (Murray and Tedrow, 1975; Murray, 2011) was initially largely concerned with the analysis of trace evidence samples by geological methods, while Geoforensics encompasses the application of all Geoscience methods in legal enquiries (Ruffell and McKinley, 2008). Recently, Forensic Geology has been redefined as “the application of geology to policing and law enforcement, which may potentially be applicable to a court of law” (Donnelly et al., 2021, p. 1), but therein applied to a wide range of investigations, encompassing ground searches for burials, crime scene examination and geological trace (sample) evidence (from which the discipline emerged).Simply put, Forensic Geology is concerned with the geologic aspects of the search, the scene and the sample: these require spatial data, be it as part of the search in the application of a Geoforensic Search Strategy (Donnelly and Harrison, 2021); the context of a crime scene (Pirrie et al., 2021; Cox and Hunter, 2005); or the location of a sample, which may form part of trace evidence (Bergslien, 2012). Our three case studies encompass exactly this tripartite division, the first being a search, the second a search that became a scene, and the third, a search that became a crime scene with samples taken.
Objectives and Methods
To create a base map of the search area required in each case study, a near-time orthoimage and digital surface model was required to overlay field-collected (spatial) geological, topographic and pertinent Geoforensic information to update published maps or to create bespoke versions applicable to forensic geology. The area incorporated was arbitrary in each case and could be extended if required. Each case had different goals (search, scene, sampling) and scales of mapping, but the need for accurate base maps at each site was the same. These studies were conducted at three locations over 4 years in response to police requests for operational assistance, with software technology progressing and drone models changing on a regular basis, from DJI (Da-Jjang Innovations, China) models including: Mavic Pro, Phantom Pro RTK (real-time kinematic), Inspire 2 (equipped with a Sentera 6X multispectral camera or Zenmuse X4S RGB camera) and Mavic 3 Multispectral RTK, all flown under an A1 and A3 UK drone licence with comprehensive insurance. The type of drone is secondary not so critical if high quality (the authors suggest a minimum of 8 megapixels) imagery can be obtained with a strong GNSS signal or ground-control points and autonomous flight with a fixed overlap, which depends on terrain type, flight altitude and processing software (Gargari et al., 2023).
In general, drones were flown at an altitude to achieve a ground sampling distance (GSD) of 3 cm/pixel or better in orthomosaics. Flight patterns were linear or crosshatch and varying camera angles and altitudes were used, depending on terrain and objectives. Where GNSS positioning during data acquisition was weak or additional accuracy was required, ground control points (GCPs) or real-time kinematic (RTK)-enabled surveys were used if possible. Flights were limited to dry weather when winds were under the threshold for safe drone flight. Drone images were uploaded to either Pix4D© (1under licence) or DroneDeploy© (2, under licence) to process images into an orthomosaic and digital surface model, requiring a minimum processing time of approximately 10 min to up to 12 h, depending on the size of the dataset. The RMSE of x, y, and z errors for the drone outputs in the case studies presented below was approximately 1 m or less.
Once completed, these outputs were exported as GeoTIFFs and either loaded onto a GNSS-enabled tablet capable of running ArcPAD or equivalent, a tablet linked to differential GNSS or real-time kinematic capabilities (such as a mobile phone connected to a base station) or printed in colour on matte paper for traditional geological mapping using hand annotation. Waypoints were then taken at points of geological/geomorphological interest or where a meaningful change was noted, and coded either by number or FGDC (Federal Geographic Data Committee, 2023) symbology. While some symbology is sufficient for field use, re-drafting is often required for readable output in reports, as shown in the maps in Figures 2, 4, 5C. Standard geological mapping then commenced, with an easy ability to switch between orthoimagery and topography. Where GNSS location was poor in valleys and under trees, surrounding waypoints were taken and compared with dead-reckoning, avoiding the need for a total station or dumpy level (surveyor’s level), both of which could have been used.
Case Studies: Background
We present three contrasting scenarios where near-time topographic base maps required geological field mapping as part of a Geoforensic Search, with all three becoming crime scenes. The first case study follows the standard protocol of assimilating available published data (geological maps) as a desktop study to inform refined field mapping. The second case study comprises a search operation in a remote location, focussed on police line searches, geophysical detection, and scent dogs where geological mapping was not anticipated as key, but became necessary while on site to assess areas of diggable thickened superficial geology (on the concept of diggability, see Donnelly and Harrison, 2013). One location of interest at this study site was then excavated by forensic archaeologists and while on site became a crime scene, with no time or access to published data, thus a drone-derived topographic base map of both the search area and scene was required. The third case study was part of a wider search and does have relevant published geological information that was accessed prior to fieldwork, but the search area that was sampled as a crime scene was only a few hundred square metres, requiring mapping at a higher resolution than available Ordnance Survey maps or aerial/satellite imagery.
Case Study 1: Wide Area Geological Mapping for Geoforensic Searches
Background and Site-Specific Methods
This location, in the northwest of Ireland, was identified as a search area by the police as part of a cold-case review. Re-visiting the site and extending the search was recommended in case the original work was overly focussed on one location within the site (Figure 1). Being a cold case (without urgency to be on site) and covering an area of 400 m × 300 m, this site was distinct from the other two case studies and offered time to conduct a desktop study of available geologic maps and publications. The forensic geology of this location was published by McKinley et al. (2009), who used topographic and geological mapping to inform sampling locations for shallow groundwater studies. However, since the publication of the above work, terrestrial search methodology has advanced considerably (Pringle et al., 2012; Donnelly and Harrison, 2017), and would have been preceded by a Desktop Study of available geological, soil, historical, topographic, aerial imagery and anecdotal information. As this study is not an account of the full Desktop Study, we only used the relevant background geological information (Figures 1A, B) to place our methodology in context.
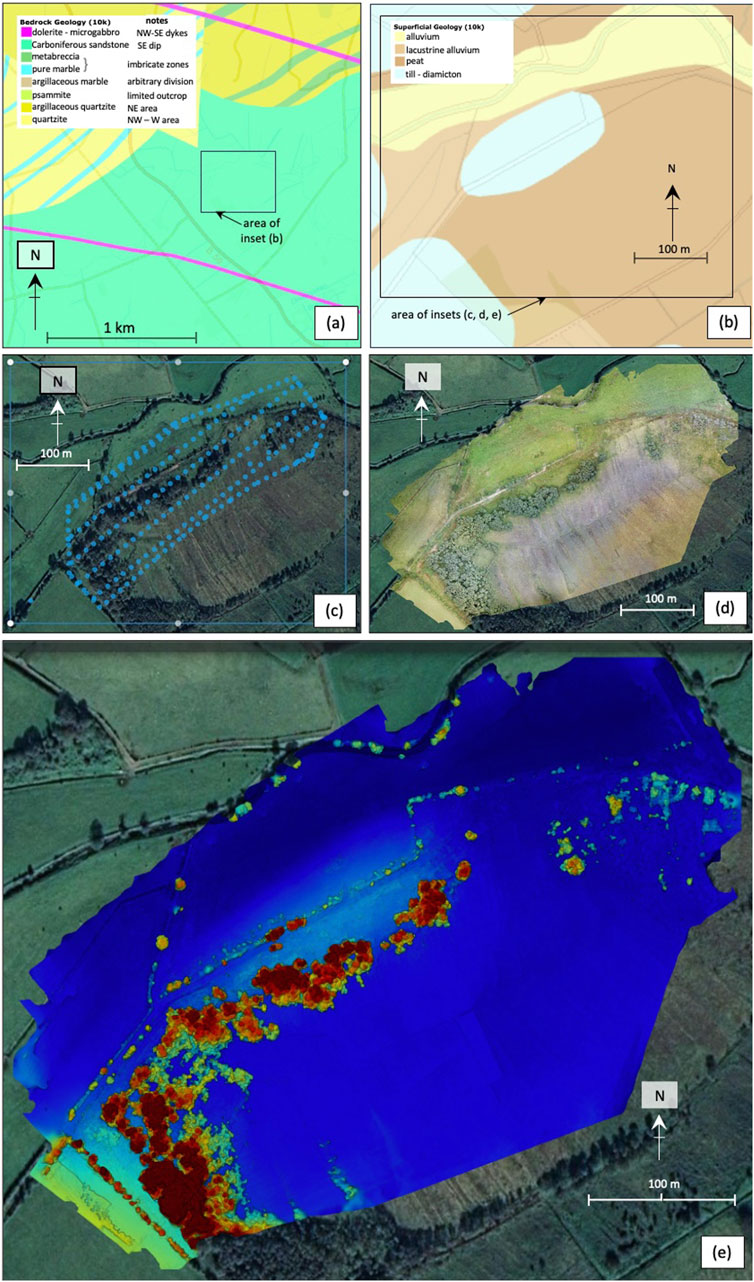
Figure 1. Data flow and outputs used for Case Study 1. (A): Bedrock geological map, showing the site as underlain by Carboniferous sandstones, with Neoproterozoic metamorphic rocks to the north and northwest (yellow and green), cut here by two Palaeogene microgabbroic dykes3; (B): Superficial geology map showing the area of inset from (A)3. (C) drone flight path and imagery locations (blue-green dots) over the area of interest at 65 m altitude, 75% front overlap and 70% side overlap processed using DroneDeploy©; (D) orthomosaic derived from images of the area of interest processed using DroneDeploy© with a ground sampling distance (GSD) of 2 cm/pixel; (E) digital surface model of the area of interest with 7.78 m/pixel GSD, with low elevation ground in blue (approximately −1 m relative altitude to take-off), and higher elevation in red (approximately 7.3 m relative altitude).
The Geological Survey of Northern Ireland (Geological Survey of Northern Ireland, 2023) shows that the bedrock at the location is underlain by Carboniferous sandstones (Claragh Sandstone Formation), with Neoproterozoic (Dalradian) metaquartzites and metalimestones (low-grade marble) to the north and northwest which were cut by NW-SE trending Palaeogene dolerite-basalt dykes (Figure 1A). The superficial geology comprises Quaternary diamicton (till), flanked and onlapped by peat and alluvium (Figure 1B). To geologically map these units together in greater detail to assess potential (diggable) burial locations, an updated base map was required. Reasonable Ordnance Survey of Northern Ireland (OSNI) maps exist for the location, but anecdotal accounts and historical imagery from Google Earth show considerable uncontrolled tree and shrub growth, altered field boundaries, peat excavation and water course alteration. For these reasons, real-time imagery was required, so an autonomous drone flight using a DJI Mavic Pro at 65 m AGL (above ground level) captured 265 images (Figure 1C) which were processed into an orthoimage (Figure 1D) and a digital surface model (Figure 1E) in GeoTIFF format. The latter was used as a geological base map to highlight geomorphological changes and features relevant to forensic geology, notably the generation of a RAG (red, amber, green) diggability map, similar in concept to the Geoforensic Search Strategy set forth by Donnelly and Harrison (2013). From these drone-derived GeoTIFFs, three critical map types were generated after incorporating field observations: geology, a digital surface model estimating geomorphology and forensic geology (Figure 2).
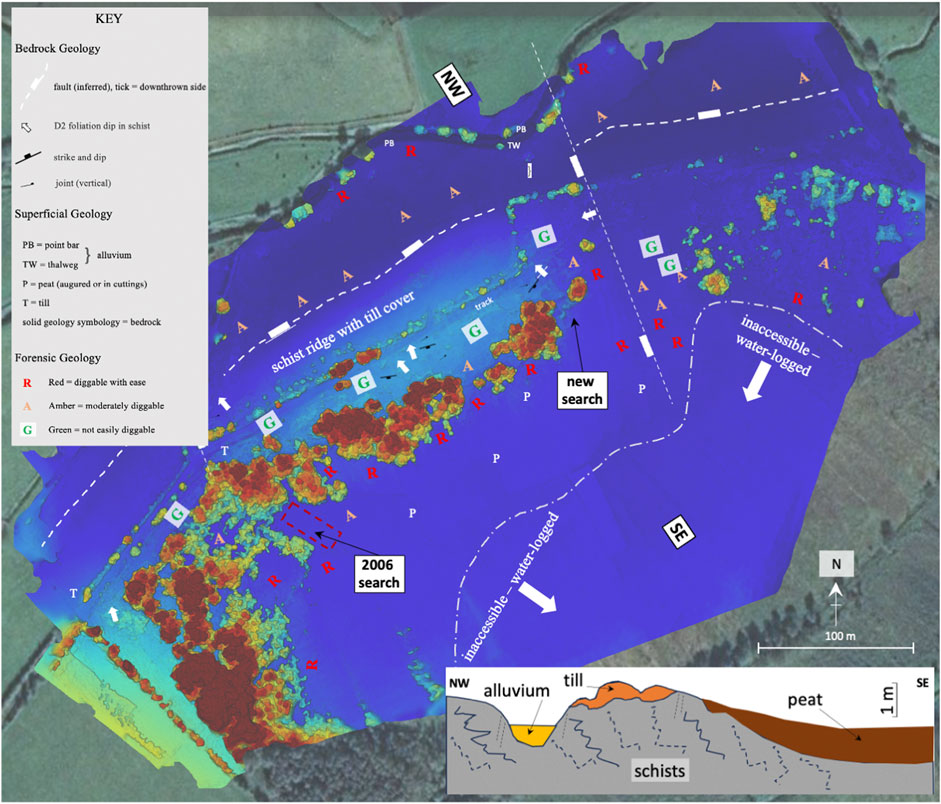
Figure 2. Bedrock and superficial geological map with forensic search information, overlain on digital topography (from Figure 1E), used in the field for Case Study 1. The basemap has been artificially made lighter for annotation visibility. The inset cartoon is a cross-section conceptual geological model from annotations “NW” to “SE” on the map, indicating the extent of (undiggable) schists and till, more diggable thin peat and soil, with diggable peat and alluvium. A highly conjectural fault is shown on the map to the north of the site, to bring older (Neoproterozoic) rocks to the surface where the Carboniferous is mapped.
Results
Structural data was input as waypoints in the field and the superficial deposits of alluvium, till (diamicton) and peat were mapped. These, combined with topography and orthoimagery allowed consideration of areas with thick, soft, superficial deposits (peat and alluvium) and translation into the green, or diggable ground, and amber or red areas - not diggable with hand tools. The original search area in 2006 (see Figure 2) comprised these attributes, combined with behavioural analysis and information from the time of the offence (the supposed abduction and murder of a teenage woman), assuming that an offender would move as far as possible along the track (labelled on Figure 2) before finding a covert and diggable location. This supposition took precedence and with corroborating features found, a more detailed search and excavation took place, with some items of interest recovered, but no human remains. The approach taken here considered previous searches and publications but used the digital output to ensure objective map coverage of the area, resulting in a second location being identified (“new search” in Figure 2). As with the 2006 search, significant items associated with both the deceased and potential offender were recovered, but no remains of the missing person (presumed murdered) were found. These findings at both search locations confirmed the search area as one of prolonged offender activity. If the area had other possible burial locations nearby, this methodology could be extended as indicated in the Methods (above), but with the river to the north and east, and impassable peat bog to the south, it appeared likely that items were buried here, some left, and some moved elsewhere. The method also shows the value of identifying this area of elevated ground as an inlier of the metamorphic rocks known to occur ∼500 m to the north, with superficial deposits covering both areas, possibly through faulting at the crest of a normal fault, a reversal north to south or a horst.
Case Study 2: Geological Mapping for Geoforensic Search and Scene
Background and Site-Specific Methods
This site was also identified through police information concerning a missing person, likely a homicide with buried remains. It contrasts with Case Study 1 however, in that there was limited previous geological mapping, apart from regional (1:635,000 scale) information. This was not considered as the Search commenced, as the information gathered indicated an almost exact spot where the burial took place in 2010, requiring confirmation from ground disturbance, including from shallow geophysics and cadaver dog indications. Background information included witness statements of the offenders leaving vehicles on a forest track (Figures 3A, B) off a minor mountain road and walking ∼250 m through a forest to the burial location, an area described as a hollow between the trees and a stream. On locating the general area, the forest had been felled for timber and re-planted, suggesting that any human remains present would have been discovered during the clearing and re-planting (see Figures 3D, E for Google Earth images from 2014 to 2020 that show the deforested site and the site at time of flight). However, less-disturbed areas remained in the approximate location of the supposed burial, as indicated by the witness. This possible burial location comprised a 50 to 100-m-wide area (average = 75 m across), extending for ∼250 m parallel to a stream, where the original pre-forestry geomorphology was preserved. Thus it became apparent that a bedrock and superficial geological map would have to be constructed, using the current topography to elucidate what was present 10 years prior to the search at the time of the supposed offence (and potentially before this) to find a hollow area of ground at about the right distance from the track. As the search and possible recovery of any human remains had already started, this had to be done in near-time. Google Earth provided confirmation of the landscape and forestry changes from 2006 to 2009 (Figures 3A, B), but without the resolution or topography required for mapping. An autonomous drone flight using a DJI Phantom with RTK positioning captured 625 images (Figure 3C) using DroneDeploy, with further detail captured along the stream using a DJI Mavic Pro. These were processed using DroneDeploy to generate a base topographic map from which an initial search area was delineated in Figure 3F according to the evidence provided (described above).
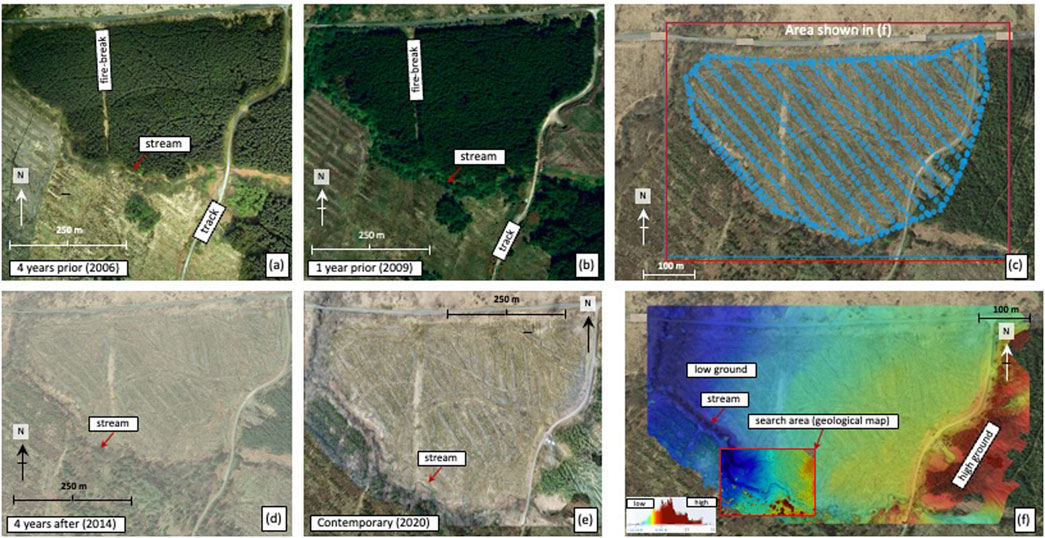
Figure 3. Background information and drone outputs for Case Study 2. (A, B) from Google Earth©, confirming the presence of managed coniferous forest in 2006 and 2009, prior to the alleged time of the incident and absent afterwards in 2014 and 2020 in (D, E). (C), location of individual images from drones, processed to create (F), the near-time topographic map in DroneDeploy© using photogrammetry.
Results
Consideration of the whole scene (Figure 3A) focussed on a 125 m × 250 m search area (see inset on Figure 3F) that was compatible with the background information, being approximately the correct distance from the minor road and forest track, adjacent to the stream and where previous conifer trees would have been sparse or absent at the time. However, even this small area presented numerous problems, due to soil excavation for sapling planting, which had to be differentiated from the natural geomorphology.
Thus, an integrated geological – geomorphological map was made (Figure 4), using the near-time topography from the drone imagery (Figures 3C, F). This was very informative as Figure 4 shows in the more focussed search area that the stream changed its course from meandering (where it was on a previously unmapped olivine microgabbro dyke), to incised. This change in fluvial form occurred in the centre of the search focus area (Figure 4). Abandoned meander channels were identified by their characteristic thalweg morphology, with remnant point bar deposits remaining, some of which had been excavated for sapling planting. The majority of meanders, however, plus the former meander bases had been infilled with peat. The combination of bedrock mapping to explain the geomorphology and superficial deposit distribution made it possible to differentiate between former natural landforms and subsequent forestry activities, which coincided with where the burial was supposed to have occurred, limited to a 20 m × 20 m grid. This area thus became a crime scene, subject to forensic archaeological excavation.
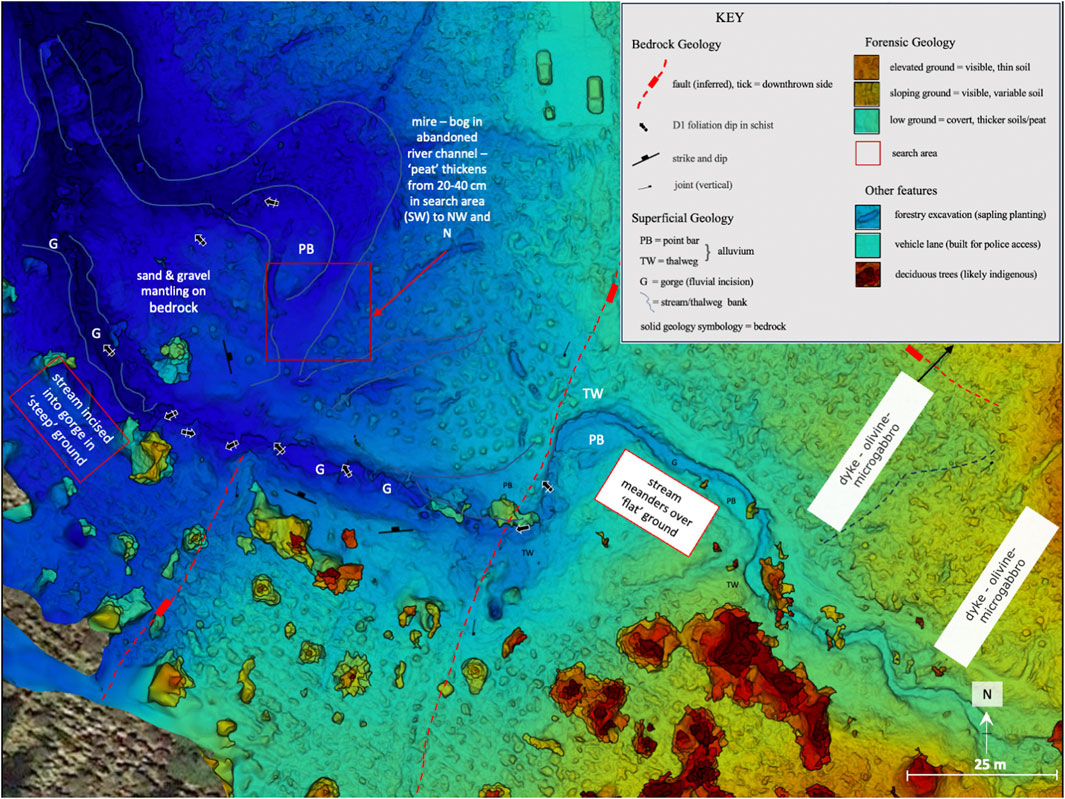
Figure 4. Combined geological, geomorphological (estimated by a digital surface model) and forensic geological map of the search area seen in Figure 3F on real-time topography. The key feature is the change in stream form (east to west) from meandering to incised at the interpreted fault in the centre of the search area. The key has forensic geological notations on the soil and thus diggability.
Case Study 3: Geological Mapping and Sampling at a Crime Scene
Background and Site-Specific Methods
This site shows the natural progression of downscaling from the wide-area search of Case Study 1, through scene identification in Case Study 2 and how the same approach can be applied at the scale of the crime scene itself. The location was subject to a search for illegal weapons hidden in near the border between the Republic of Ireland and Northern Ireland (UK), where suspect vehicles had been known to stop via access to car GNSS telemetry taken from seized vehicles when suspects were arrested. Two questions remained: where to search within the site, and what soil samples to take.
Both questions were answered using near-time drone imagery as background mapping information. A DJI Mavic Pro was used to gather 92 images (a dense grid not shown) over the site, which were processed in DroneDeploy© to produce an orthomosaic of the search areas (Figure 5A) and topography for Geoforensic assessment (Figure 5B), and then geological mapping for integration with access routes and thus sampling (Figure 5C).
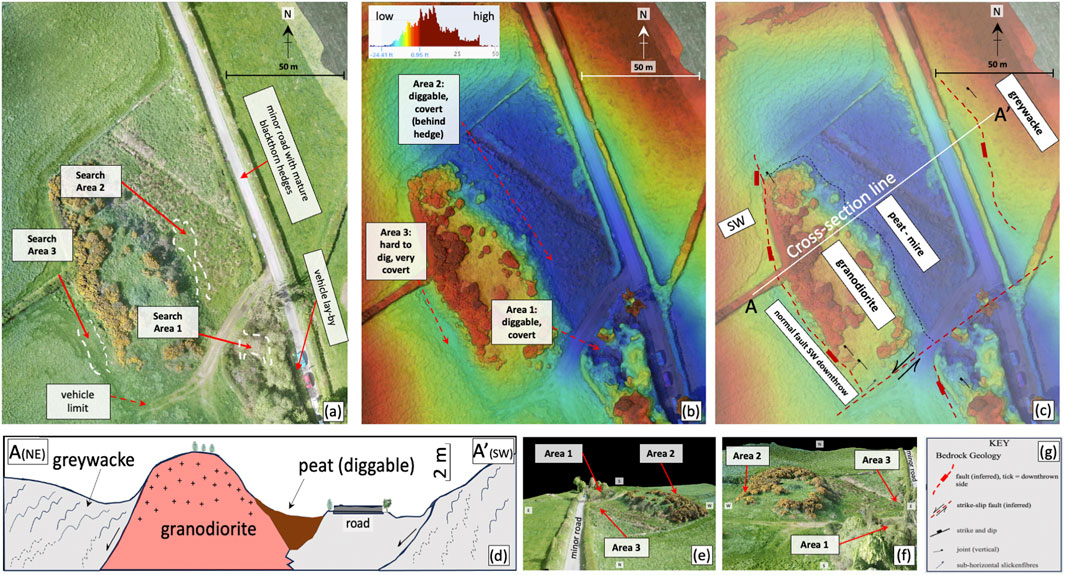
Figure 5. Imagery and geological information/interpretation for Case Study 3 – (A) the final part of the search, through to the scene shown in (B) and thus geologically-informed sampling in (C) [see the key to this map in (G)]. (D) conceptual cross-section indicating the limits of where material might be buried (diggable peat). The figure is modified from Ruffell et al., 2023). (E, F) show screenshots of the 3D model generated from drone imagery, which was provided to allow search personnel a bird’s eye view ahead of searching the area. Data processing was done via DroneDeploy©.
Results
Search personnel identified three potential areas that were covert and could allow digging and the burial of a weapons cache (Figure 5A). As this work proceeded the drone topographic map (Figure 5B) was used to evaluate both this decision and to construct a combined bedrock and superficial geological map (Figure 5C), for soil and sediment sampling. The drone-derived topographic map effectively mimics a red-amber-green (or RAG) diggability map with blue topographic lows representing diggable land (as opposed to green) but showed that without the initial evaluation by security personnel on site, a Geoforensic approach would have produced (rapidly derived) similar results. Geological mapping showed the bedrock geology to be a faulted block of granodiorite, juxtaposed against Lower Palaeozoic greywackes, with peat deposits in a mire, developed in the half-graben (Figures 5C, D). Soil samples taken at the access points were augmented by samples taken further into the search area, which may have come into contact with the suspects, but still served as local controls for soil variation, especially useful as suspects reported simply stopping on the road for innocent reasons. An arms cache of four assault rifles, five machine pistols and two handguns were located and retrieved in Area 1 (Figure 5B), and one suspect was charged with a range of terrorism-related offences, with the soil samples from the track to the “vehicle limit” shown on Figure 5A forming one element of the evidence in the case.
A Possible Workflow for Creating Digital Geoforensic Maps
A generic workflow is shown in Figure 6, with mapping objectives and delineation of the area of interest as the first two steps, as this will dictate the size of the study area, the drone requirements and the processing needed to generate up-to-date high resolution orthoimagery and topography. The authors suggest an in-person site evaluation to determine flight hazards such as power lines or unexpected human activity and to confirm the area of interest. In preparation for an autonomous drone survey, appropriate licences and authorisations are essential, as are considerations for battery capacity and on-site charging if necessary. There are dozens of flight planning applications available on mobile devices and drone controllers, and we have had success using DroneDeploy and DJI GS Pro. Processing software depends on budget, dataset size, desired outputs and access to the internet. Common cloud- and software-based applications are listed in Figure 6, Step 5. Once processing is complete, outputs can then be exported to a GNSS-enabled tablet or printed for field annotation, which, once completed, result in a final up-to-date, high-resolution map with all the necessary information to conduct a Geoforensic search.
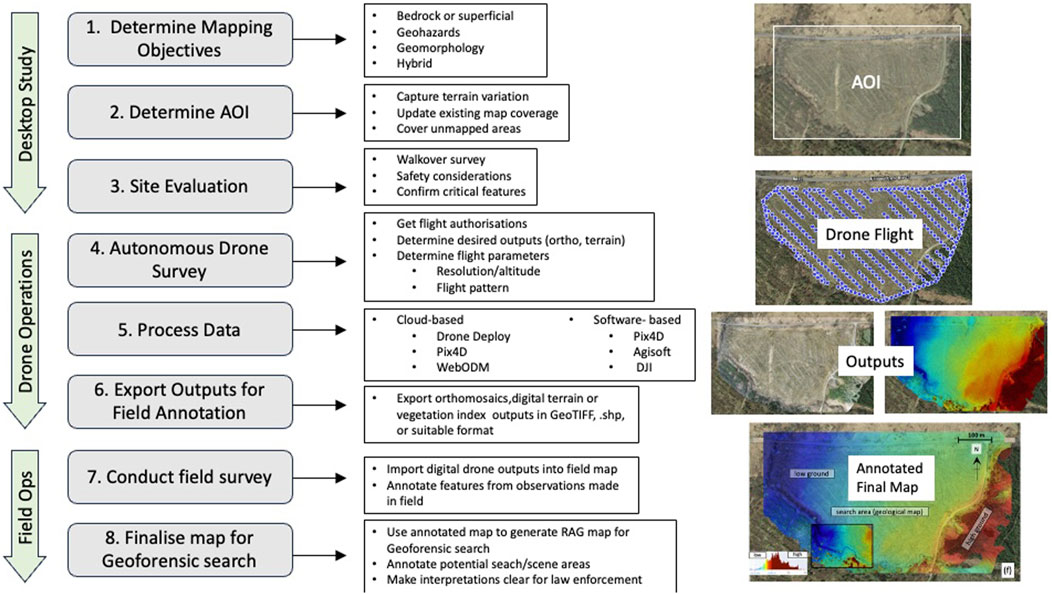
Figure 6. Eight-step generic workflow for the generation of an up-to-date high resolution annotated Geoforensic search map using autonomous drone flight.
Conclusions and Recommendations
The three case studies described are examples of a larger number of near-time geological mapping procedures that we have carried out for Geoforensic casework, although the use of such mapping extends beyond forensics. These include the need for contemporaneous maps of landslips, bog slides, foreland bedrock (exposed after glacier-melt) and storm-stripped shingle beaches. All use the same principles developed here for forensic-based work at different scales, from wide-area searches (∼500 m × 500 m) or greater, as in Case Study 1, through a focussed crime scene scale (∼5/10 m–20 m) in Case Study 2, to provide search and sampling information at the crime scene itself (Case Study 3).
We suggest in all three case studies that the creation of near-time maps for annotation in the field is invaluable in focussing the Search to Scene and documentation of the crime scene itself. The use of off-the-shelf drones that capture a scene using autonomous flight can be done with simple training and inexpensive equipment, although photogrammetry software can be expensive unless freeware, such as Open Drone Map is used. Processing times for small areas with few photos can be minimal, and with sufficient mobile signal can be uploaded to cloud processors in real-time without the need for a field laptop.
A workflow for how to progress a search area from desktop study to drone flight to the final field map is laid out in Figure 6, where further considerations are outlined such as flight altitude, overlap, processing parameters and exports. These exports, often in the form of GeoTIFFs, can be uploaded to GIS software on a tablet and used as an up-to-date base map for annotations in the field, which has proven advantageous in Geoforensic Searches.
Data Availability Statement
The datasets presented in this article are not readily available due to anonymisation of location. Requests to access the datasets should be directed to YS5ydWZmZWxsQHF1Yi5hYy51aw==.
Author Contributions
First manuscript including Figures 1–5 contributed by author AR. BR did extensive review and added Figure 6 and wrote the section regarding the proposed workflow and also added own words to conclusions, and own words throughout as necessary. BR did work to adjust figures, originally created by AR. All authors contributed to the article and approved the submitted version.
Funding
The author(s) declare that no financial support was received for the research, authorship, and/or publication of this article.
Conflict of Interest
The authors declare that the research was conducted in the absence of any commercial or financial relationships that could be construed as a potential conflict of interest.
Publisher’s Note
All claims expressed in this article are solely those of the authors and do not necessarily represent those of their affiliated organizations, or those of the publisher, the editors and the reviewers. Any product that may be evaluated in this article, or claim that may be made by its manufacturer, is not guaranteed or endorsed by the publisher.
Acknowledgments
Case Study 1 was developed through long and detailed discussions with staff at Queen’s University, Belfast (Wolfram Meier-Augenstein, Jennifer McKinley, Helen Kemp) and the Police Service of Northern Ireland (John Gilmore, Raymond Murray, Trevor Cooke, Stephen McIlory). Case Study 2 was instigated by staff from Police Scotland (Brian Geddes, Suzanne Chow) and was greatly enhanced by fieldwork and discussions with Lorna Dawson (James Hutton Institute). Numerous un-named security personnel assisted at the Case Study 3 site.
Footnotes
3All rights reserved. Topographic material is Crown Copyright and is reproduced with the permission of Land and Property Services under delegated authority from the Controller of Her Majesty’s Stationery Office, © Crown Copyright and Database Rights (2023) MOU577.3. © Crown Copyright and Database Right 2023, © Ordnance Survey Ireland - SpatialNI provided by Ordnance Survey of Northern Ireland®.
References
Bemis, S. P., Micklethwaite, S., Turner, D., James, M. R., Akciz, S., Thiele, S. T., et al. (2014). Ground-based and UAV-Based Photogrammetry: A Multi-Scale High-Resolution Mapping Tool for Structural Geology and Paleoseismology. J. Struct. Geol. 69, 163–178. doi:10.1016/j.jsg.2014.10.007
Bergslien, E. (2012). An Introduction to Forensic Geoscience. Chichester, Sussex, UK: John Wiley & Son, 363p.
Cox, M., and Hunter, J. (2005). Forensic Archaeology: Advances in Theory and Practice. London: Routledge, Taylor & Francis Group, 233pp.
Crillio, D., Cerritelli, F., Agostini, S., Bello, S., Lavecchia, G., and Brozetti, F. (2022). Integrating Post-processing Kinematic (PPK)-structure-from-motion (SfM) with Unmanned Aerial Vehicle (UAV) Photogrammetry and Digital Field Mapping for Structural Geological Analysis. Int. J. Geoinformation 11, 1–25. doi:10.3390/ijgi11080437
Donnelly, L. J., and Harrison, M. (2013). “Geomorphological and Geoforensic Interpretation of Maps, Aerial Imagery, Conditions of Diggability and the Colour-Coded RAG Prioritization System in Searches for Criminal Burials,”in Environmental and Criminal Geoforensics Editors D. Pirrie, A. Ruffell, and L. Dawson (London: Geological Society), 384, 173–194. doi:10.1144/sp384.10
Donnelly, L. J., and Harrison, M. (2017). Ground Searches for Graves and Buried Targets Related to Homicide, Terrorism and Organised Crime. Episodes 40, 106–117. doi:10.18814/epiiugs/2017/v40i2/017012
Donnelly, L. J., and Harrison, M. (2021). “Chapter 3. Geoforensic Search Strategy (GSS): Ground Searches Related to Homicide Graves, Counter Terrorism and Serious Organized Crime,” in A Guide to Forensic Geology. Editors L. J. Donnelly, D. Pirrie, M. Harrison, A. Ruffell, and L. Dawson (London: Memoirs of the Geological Society), 21, 44–136.
Donnelly, L. J., Pirrie, D., Harrison, M., Ruffell, A., and Dawson, L. (2021). in A Guide to Forensic Geology (London: Memoirs of the Geological Society), 21, 217p.
Federal Geographic Data Committee (FGDC) (2023). Geospatial Files for Geologic Maps. Available at: https://www.geoplatform.gov/(Accessed December 15, 2023).
Gargari, A. M., Ebadi, H., Esmaeili, F., and Latifzadeh, S. (2023). Dynamic 3D Network Design for UAV-Based Photogrammetry in Mountainous Terrain. Environ. Earth Sci. 82, 188. doi:10.1007/s12665-023-10864-9
Geological Survey of Northern Ireland (2023). Northern Ireland GeoIndex. Bedrock and Superficial Deposits. 1:10,00 and 1:50,000. Keyworth, Nottingham: British Geological Survey. Available at: https://mapapps2.bgs.ac.uk/gsni_geoindex/home.html.
Houck, M. M., and Siegel, J. A. (2010). The Fundamental of Forensic Science. Kidlington, Oxford, UK: Elsevier, 363p.
Jones, R. R., McCaffrey, K. J. W., Wilson, R. W., and Holdsworth, R. E. (2004). “Digital Field Data Acquisition: Towards Increased Quantification of Uncertainty during Geological Mapping,”in Geological Prior Information: Informing Science and Engineering. Editors A. Curtis, and R. Wood (London: Geological Society, London, Special Publications), 239, 43–56. doi:10.1144/gsl.sp.2004.239.01.04
McKinley, J., Ruffell, A., Harrison, M., Meier-Augenstein, W., Kemp, H., Graham, C., et al. (2009). “Spatial Thinking in Search Methodology: A Case Study of the ‘No Body Murder Enquiry’. West of Ireland,” in Criminal and Environmental Soil Forensics. Editors K. Ritz, L. Dawson, and D. Miller, 285–302p.
Murray, R. C. (2011). Evidence from the Earth: Forensic Geology and Criminal Investigation. 2nd ed. 240p. Missoula, MT USA: Mountain Press Publishing.
Murray, R. C., and Tedrow, J. C. (1975). Forensic Geology: Earth Sciences and Criminal Investigation. New York, USA: Rutgers University Press, 217p.
Nesbit, P. R., Durkin, P. R., Hugenholtz, C. H., Hubbard, S. M., and Kucharcysk, M. (2018). 3-D Stratigraphic Mapping Using a Digital Outcrop Model Derived from UAV Images and Structure-From-Motion Photogrammetry. Geosphere 14, 2469–2484. doi:10.1130/ges01688.1
Pirrie, D., Ruffell, A., Dawson, L., and McKinley, J. (2021). “Chapter 4: Crime Scenes: Geoforensic Assessment, Sampling and Examination,” in A Guide to Forensic Geology. Editors L. J. Donnelly, D. Pirrie, M. Harrison, A. Ruffell, and L. Dawson (London: Memoirs of the Geological Society), 21, 137–175.
Pringle, J. K., Ruffell, A., Jervis, J. R., Donnelly, L., McKinley, J., Hansen, J., et al. (2012). The Use of Geoscience Methods for Terrestrial Forensic Searches. Earth Sci. Rev. 114, 108–123. doi:10.1016/j.earscirev.2012.05.006
Ruffell, A., Rocke, B., and Powell, N. (2023). Geoforensic Search to Crime Scene: Remote Sensing, Geophysics and Dogs. J. Forensic Sci. 110. doi:10.111/1556-4029.15293
Sikakwe, G. U. (2023). Mineral Exploration Employing Drones, Contemporary Geological Satellite Remote Sensing and Geographical Information System. GIS Procedures: A Review. Remote Sens. Appl. Sci. Environ. 31, 100988. doi:10.1016/j.rsase.2023.100988
Keywords: geoforensic search, digital mapping, drones, forensic geology, bedrock/superficial mapping, remote sensing, desktop study
Citation: Rocke B and Ruffell A (2024) Near-Time Digital Mapping for Geoforensic Searches. Earth Sci. Syst. Soc. 4:10106. doi: 10.3389/esss.2024.10106
Received: 12 January 2024; Accepted: 23 October 2024;
Published: 15 November 2024.
Edited by:
Richard O’Brien, Atkins, United KingdomCopyright © 2024 Rocke and Ruffell. This is an open-access article distributed under the terms of the Creative Commons Attribution License (CC BY). The use, distribution or reproduction in other forums is permitted, provided the original author(s) and the copyright owner(s) are credited and that the original publication in this journal is cited, in accordance with accepted academic practice. No use, distribution or reproduction is permitted which does not comply with these terms.
*Correspondence: Benjamin Rocke, YnJvY2tlMDFAcXViLmFjLnVr
†ORCID: Benjamin Rocke, orcid.org/0000-0003-2003-1392; Alastair Ruffell, orcid.org/0000-0001-6072-501X