- 1Centre for Subsurface Imaging, Institute of Hydrocarbon Recovery, Universiti Teknologi PETRONAS, Seri Iskandar, Malaysia
- 2Craytive Technologies, Vlaardingen, Netherlands
The recent proliferation of Extended Reality (XR) applications in geoscience education and research has opened new avenues for the enhanced visualization and analysis of the Earth’s geodata. This study specifically explores the benefits for teaching when supplementing industry standard software packages, such as Paleoscan, Petrel, and JewelSuite, with 3D visualization in XR. The teaching focuses on but is not limited to an understanding of subsurface seismic and well data. During this study, the undergraduate Petroleum Geoscience students transitioned from 2D computer screen visualizations to immersive XR tools. The dataset selected for teaching focuses on the subsurface carbonate EX field in the South China Sea. The EX-field in Central Luconia is located 100–300 km from Sarawak’s coastline in water depths of 60–140 m. It includes a post-stacked time- 3D seismic cube linked to wells, allowing students to work with seismic data, adjust scales, and conduct preliminary seismic analysis. The findings revealed a significant improvement in respondents’ skills in comprehending and analysing seismic and core data, enhancing the overall learning experience in Petroleum Geoscience. This paper also examines the students’ feedback on their learning experiences during virtual subsurface visualization throughout their university degree in geoscience. For evaluating learning success, we used an approach that merges quantitative and qualitative data, The students’ perceptions were assessed through anonymous quantitative surveys and questions. The analysis of student responses emphasizes the valuable learning experience offered by 3D virtual environments designed for realistic first-person navigation and freedom of movement, like a real field experience. The results highlight the potential of virtual subsurface visualization for imparting essential skills to geosciences.
Introduction
Spatial computing and extended reality (XR) visualization, like Virtual Reality (VR) and Augmented Reality (AR) have recently been applied in learning, scientific investigation, and communication. The integration of XR in geoscience has particularly thrived because of the accessibility of geodata that was once hard to reach, proving to be an effective tool for visualizing and analyzing the Earth’s geodata (Janiszewski et al., 2020; Rossi et al., 2017; Schmitz et al., 2019). Virtual reality tools offer educators and trainers the chance to tailor the learning journey and guide students through virtual tours of places that may be inaccessible in person. (Roemmele and Pantazes, 2023). The success of gaining geoscience learning advantages in terms of thinking, professional growth, and investigation is thought to be influenced by the existence, engagement, and ease of access in a virtual field experience. (Moysey and Lazar, 2019).
This study focuses on educational and learning activities in XR using the commercial extended reality or spatial computing platform for geoscience BaselineZ by Craytive Technologies BV. Solutions, 2023. The platform allows for the visualization of and collaboration around 3D subsurface geodata. This type of 3D subsurface data is commonly visualized and analyzed on 2D computer screens, with industry-standard subsurface software packages such as Paleoscan by Ellis (2019), Petrel by Schlumberger (2019), and JewelSuite by Baker Hughes (2022). The students in the study who were introduced to XR had a background in BSc Petroleum Geoscience and were familiar with software packages that visualize geoscience data on 2D screens. This study evaluates the effectiveness of immersive virtual reality tools for teaching and for gaining an understanding of subsurface seismic datasets. The authors aim to introduce XR case study teaching, complementing the typical lecture format in educational settings (Soto et al., 2023). This highlights the indispensable role of XR as a pedagogical tool, enabling instructors to bring subsurface datasets into the classroom, particularly crucial when circumstances delay physical visits to study sites like for instance to core centers. The study analyses the qualitative and quantitative impact of immersive virtual reality in the realm of geological exploration, with a specific focus on subsurface geo-data. The driving influence behind this survey lies in the imperative to derive greater value from existing geoscience models and datasets for training purposes.
Presently, differences in data at various scales, encompassing core, log, seismic, and geological models, pose a prevalent challenge. Common issues such as accessibility barriers in remote locations and underutilized assets further compound these difficulties. In addition, the conventional visualization of 3D data on 2D computer screens introduces a perceptual gap. In recognizing these complex challenges, this study considers logistical obstacles, including the remote location of the data and the necessity for simultaneous training and collaboration across diverse physical locations. Through the integration of immersive virtual reality, researchers endeavor to overcome these challenges and aim not only to bridge gaps but also enhance the overall effectiveness of geological exploration training.
This analysis examines perceptions of virtual reality implementation concerning geophysical and core data in the teaching and learning activities of graduate students at Universiti Teknologi Petronas.
Methodology and Dataset
Student Group and Teaching Goals
Altogether 25 participants engaged in undergraduate studies at Universiti Teknologi Petronas actively took part in this study. In addition to their normal course schedule the students were given the opportunity to use XR technologies for their studies. We then evaluated their experiences qualitatively and quantitatively using questionnaires.
The primary aim of our survey aligned with the educational objective of assessing students’ proficiency in recognizing and manipulating seismic data and modelling within the framework of the Seismic Methods course. Notably, the majority of the participants brought valuable prior experience with subsurface data to the table, enhancing the depth and diversity of insights in our exploration of this subject matter.
Training Dataset: Ex Field, Malaysia
The dataset we chose to be visualized in the virtual environment comprised a 3D seismic survey linked to wells. Seismic data are of vital importance in characterizing the subsurface, may it be for the development of oil and gas fields, as for monitoring oil or gas production or carbon capture and storage (CCS). Given its significance to industry, graduate students in geoscience must possess a solid understanding of seismic data. A profound grasp of the expanding role of seismic technology and industry exploration enhances the employment prospects of fresh graduates and facilitates their effective integration into any geoscience team (Onajite, 2013).
The EX Field, situated in the southern part of the province with water depths ranging from 60 to 140 m, presents a significant geological setting. Specifically, the study area, located 145 km NNW of Bintulu, Malaysia features water depths between 190–250 ft (Figure 1). Notably, a pinnacle structure covers gas buildup in a structural platform type, as highlighted by (Epting, 1980; Gartner et al., 2004; Rankey et al., 2019; Zampetti, 2010; Jiménez Soto et al., 2022).
This study strategically utilizes a comprehensive regional post-stacked migrated 3D seismic dataset, with a primary focus on EX carbonate build-up. The dominant frequency at the examined depth was 1700 m/s, as determined from the well data for the sequence of importance (Figure 2). The exploration involves recognizing nine main horizons by meticulously following clear and continuous reflectors and utilizing automatic tracking in Paleoscan software. The seismic data revealed distinct features of the EX buildup, with high-amplitude reflections in the range of 40–60 ms, accompanied by low-amplitude reflections in the overlying shale at 15–20 m intervals. The reflection domain limits exhibit sharp borders, causing sudden dips and amplitude shifts. This study meticulously explores the nuances of the northern and southern sides of the EX buildup, providing detailed insights into slope angles and variations.
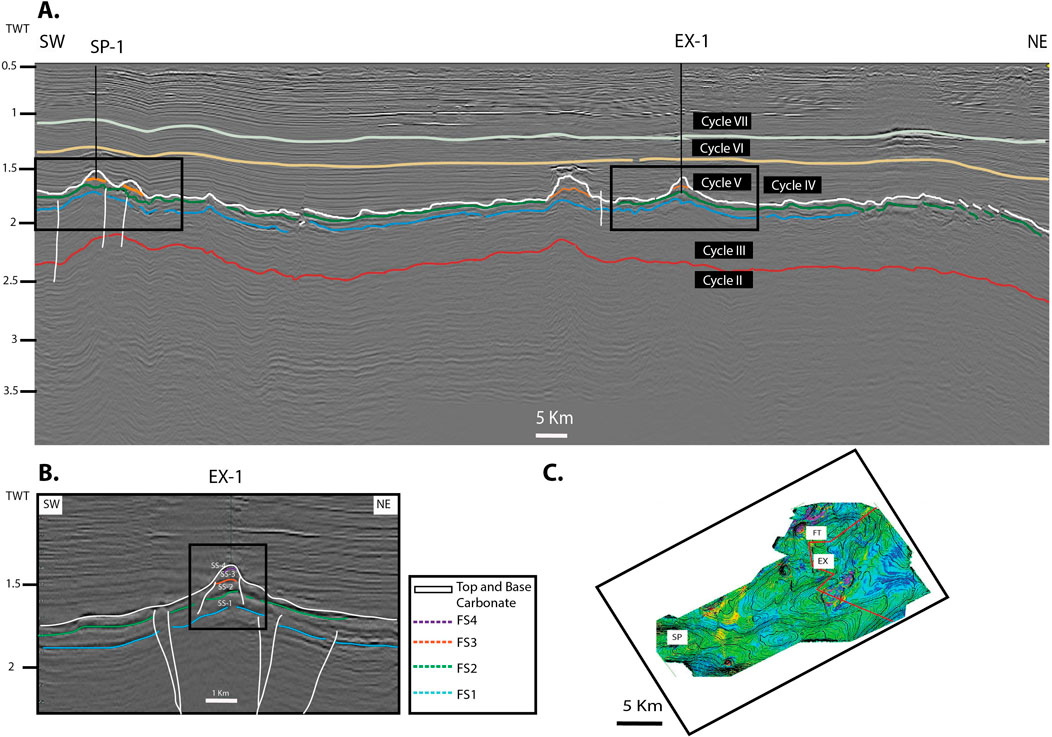
Figure 2. (A) Regional sequence stratigraphic interpretation of the EX Field. (B) Detailed image of the EX Field. (C) EX Structure in detail, top of the carbonate regional map with SP, EX and FT Fields.
Stratigraphy analysis identified distinct lithofacies and microfacies within the EX field. These include shale, mudstone/wackestone, wackestone-packstone, packstone (coral/red algae/foraminifera), packstone-grainstone (coral/red algae/foraminifera), floatstone (coral/red algae), rudstone (coral/red algae/foraminifera), and framestone (in-situ coral). Each lithofacies provided valuable insights into the environmental conditions, energy levels, and composition of the EX Field. (Figure 3).
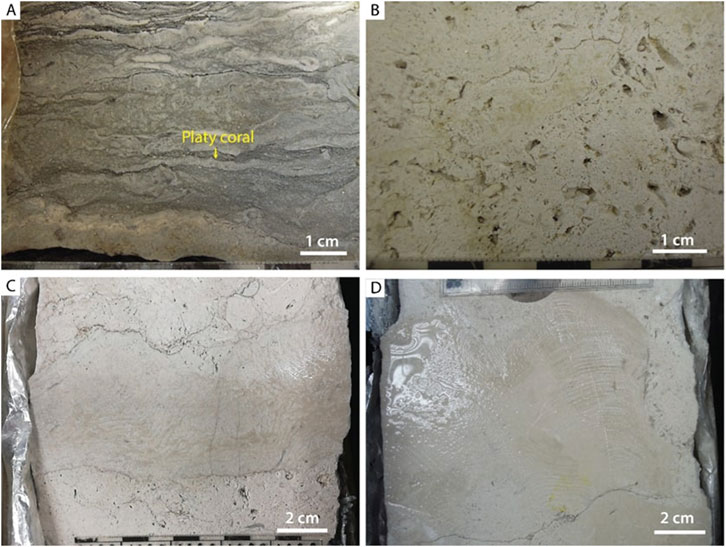
Figure 3. Lithofacies in the EX Field: (A) Floatstone (coral/red algae), (B) Packstone-grainstone (coral/red algae/foraminifera), (C) Rudstone (coral/red algae/foraminifera), and (D) Framestone (in-situ coral).
The Spatial Computing Platform
In contemporary contexts marked by rapid technological advancements, more sophisticated collaborative modalities have been unveiled. Notably, extended reality such as virtual reality and augmented reality (AR) methodologies have been developed during the last few years, transcending the constraints of the physical realm. The virtual field by (Milgram and Kishino, 1994) describes the range of experiences and helps to put the different terms in context. It describes the transition between the real environment on the one side and the completely virtual environment on the other side, The recently used term spatial computing on the other hand is somewhat like the term extended reality but emphasizes the aspect of interaction of humans with the virtual objects in the real or virtual environment.
In this paper we use the term virtual reality (VR) for closed headsets that immerse the user in a completely virtual world (VR headset). Mixed reality (MR) stands for headsets with a direct see-through vision for example Microsoft Hololens 2 by Microsoft (2019) or camera pass-through vision like the Meta Quest 3 by Meta (2023) or Apple Vision Pro by Apple (2024). Virtual objects blend with reality. In our geoscience context, users will locate themselves in their real environment (e.g., office or outdoor) while the virtual geological models, annotations or supplemental data or objects are embedded and appear as if they were there. The term augmented reality (AR) is used for tablets and phones showing the real world through the camera on the display augmented with the virtual objects. Lastly, virtual displays on (large) PC screens, or more immersivity in physical 3D visualization centers create XR experiences.
Users of XR or spatial computing can experience all objects from their personal perspective which leads to a true three-dimensional vision. Collaboration between users on different XR devices is facilitated. Each user can view the virtual content simultaneously and from their own viewpoint. In addition, interaction with the virtual content is enabled. So XR collaboration enables users to experience an asymmetrical interaction environment (Lee and Yoo, 2021).
The spatial computing platform we have used during the study, BaselineZ supports all the types of spatial computing described above (Figure 4). The platform is designed for collaboration and learning in the context of geoscience data. 3D geoscience data and supplemental data such as videos, images and documents can be securely transitioned from viewing on standard 2D screens to 3D extended reality (XR). The core of the application is the BaselineZ cloud which is powered by Microsoft Azure. The BaselineZ web client connects to the cloud and is used for user, data, and project management. Users will create projects and send their models and data from the PC to the cloud. This is either done by add-ins (plug-ins) for standard geomodelling software such as Petrel or JewelSuite, or via dedicated BaselineZ apps. Different XR clients can retrieve the data from anywhere and visualize on mobile devices (Android and iOS tablets and phones), head mounted XR devices (Meta Quest 2, 3, Quest Pro, HTC VIVE, Microsoft HoloLens 2), PC, or in visualization centers including Igloo Vision (Igloo Vision, 2023), and Barco Cave (Barco NV) systems (Barco, 2023).
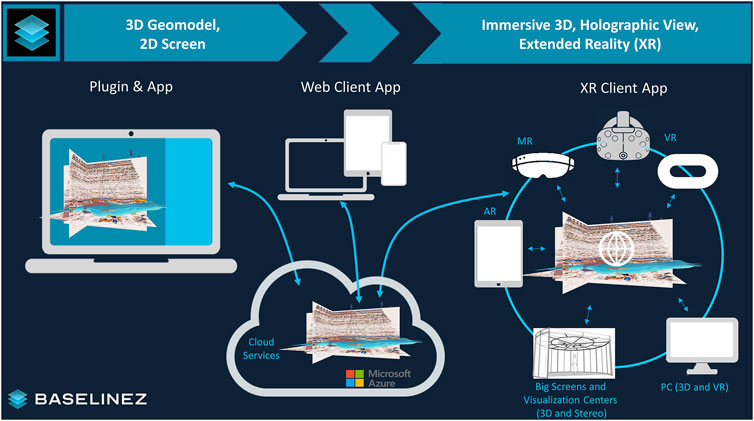
Figure 4. Concept of the spatial computing platform BaselineZ. Geoscience data that is commonly visualized by desktop geomodelling packages on 2D screens can be pushed via a plugin or app to the BaselineZ secure cloud. Projects and data can be received, and collaboratively visualized, and worked with in extended reality, using different XR clients. A web client connects to the cloud, to manage projects and data.
For our teaching experience we have worked with VR (Meta Quest 2), AR (diverse iOS and Android phones and tablets) and larger PC screens.
Each student user experienced real-time interaction with 3D virtual objects. This allowed for interactive and immersive visualization experiences. The virtual elements such as, cores, wells, surfaces, and geological model grids closely emulate reality, allowing students to engage in actions such as holding, rotating, panning, zooming in, and zooming out of objects. Similarly, properties like vertical depth can be displayed on surfaces.
Creating a Virtual Dataroom
To facilitate the training, we have developed immersive virtual environments utilizing the multiscale dataset introduced and detailed above. Employing the BaselineZ Add-In to JewelSuite, we transferred the subsurface data like seismic, horizons and wells to the BaselineZ cloud. Core data of one well and supplementary information including figures and reports have been added.
We have provided an immersive XR 3D experience designed to enhance teaching by incorporating virtual geodata data rooms and immersive 3D narratives called 3D Stories:
• Virtual Data Rooms: These environments contain the entire dataset, allowing users to interact freely with all items within the virtual space. Users can explore and manipulate multiscale data, including core samples, seismic data, figures, and standard presentations, all within a contextual 3D environment.
• 3D Stories: These narratives guide users through the dataset in a structured sequence, similar to traditional slide presentations but with enhanced interactivity. Interaction within the 3D stories is purposefully limited compared to the full capabilities of the virtual data rooms. This design choice facilitates user engagement and ensures a coherent narrative flow, guiding the user through the data and geoscience story. Users retain the ability to select and change their position in the room and interact with all objects. Stories can be either auto-narrated with prerecorded content or presented live by a presenter within the virtual room. This immersive approach not only enhances user engagement but also provides a comprehensive and interactive way to present complex datasets and geoscience stories.
Subsequently, these data rooms and stories were retrieved and visualized by student users (Figure 5).
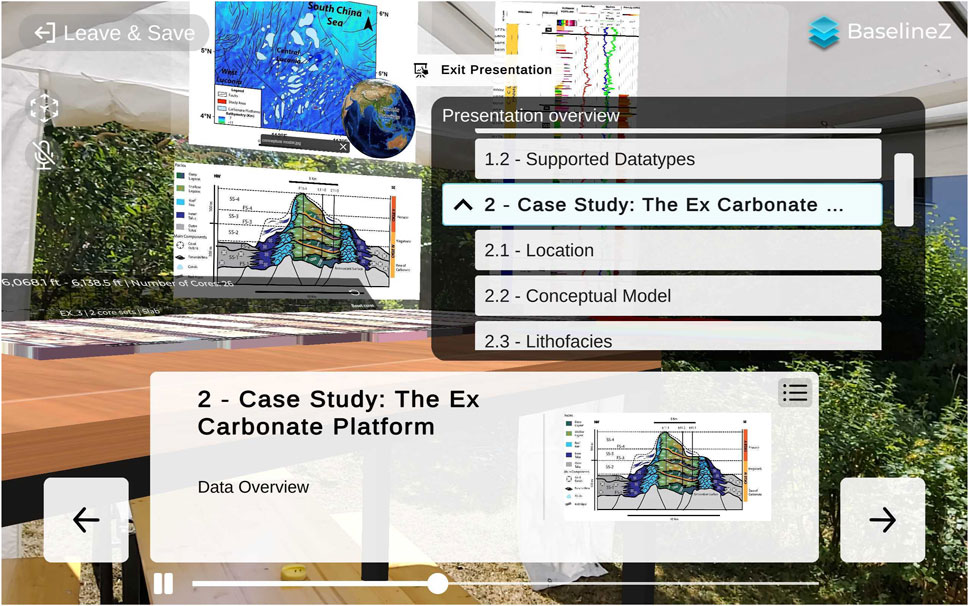
Figure 5. 3D Story as seen on an AR tablet device – here in an outdoor environment. The User Interface shows the Story in presentation mode the same as the students experience it. The teachers have prepared immersive lessons, incorporating 3D models, charts, and holograms. The audio recording feature enhances accessibility, allowing students to revisit the lessons at their convenience, and provides flexibility and continual access to geoscience content for students.
Our proposed XR data room introduced a collaborative workspace (Figure 6) that integrates virtual objects, enriching engagement across both dimensions. Students in this extended reality (XR) workspace can personalize their interaction environment by selecting from 3D, virtual reality (VR), or augmented reality (AR) options, tailoring the collaborative experience (Lee and Yoo, 2021).
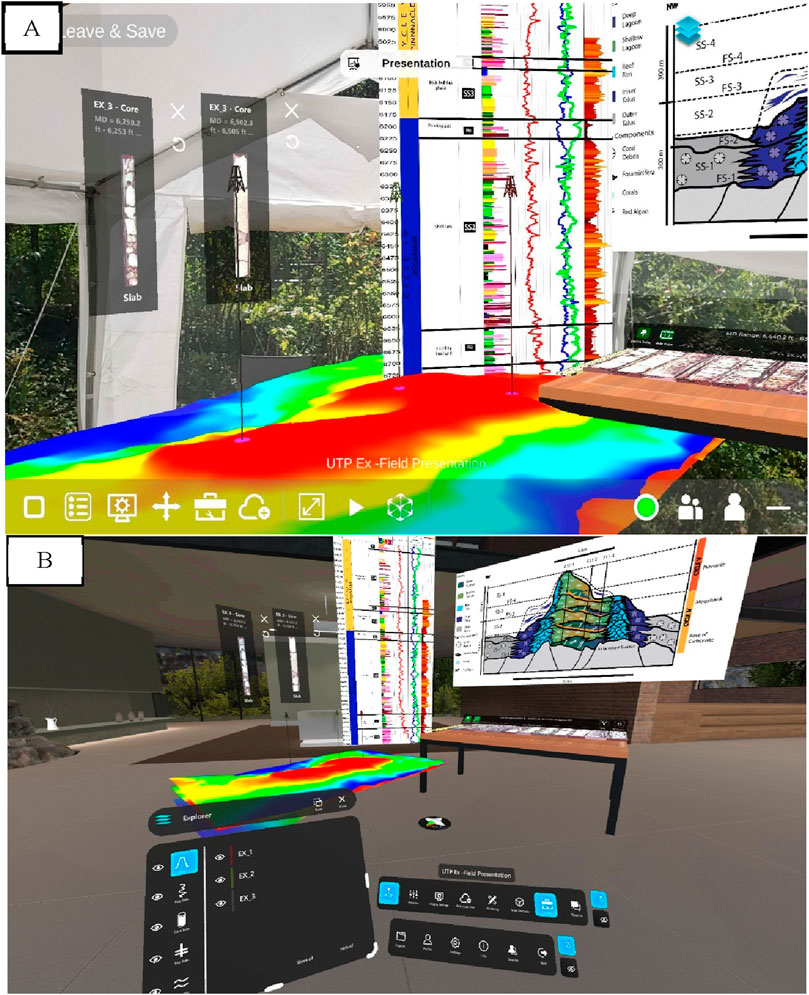
Figure 6. (A) Example for a geoscience dataroom visualization on a tablet (example outdoor experience) and (B) a VR headsets like Quest 2. The students can simultaneously explore core data and well-core analysis. The XR platform supports merging theory with real-world geological data and offers a transformative learning experience in VR.
Setup of the Study
The initial phase of our study involved students from Universiti Teknologi Petronas located in Seri Iskander, Malaysia during the 2022–2023 academic year, specifically focusing on undergraduates in their final year of the geology program. This group of students was collectively skilled at utilizing desktop software for seismic and well-log visualization. In the initial stage, we meticulously designed questionnaires aimed at assessing their familiarity with subsurface geodata and their pre-existing exposure to virtual reality.
Once we completed this foundational analysis, the second phase started with the introduction of BaselineZ within the extended reality (XR) environment. Following this standard organization, participants were actively engaged in XR geoscience sessions, comprising both virtual reality (VR) and augmented reality (AR). These sessions facilitated the student’s ability to navigate through 3D seismic cubes, well logs, and digital cores, with each VR session extending over 10–15 min.
Within this immersive framework, participants, equipped with headsets, were provided instructions to adjust object sizes, manipulate color sets, and conduct preliminary seismic interpretations. This interactive engagement acted to foster data enhancement and enrichment. The dataset itself comprised seismic cubes, well logs, and digital cores, thereby amplifying virtual reality experience.
After the immersive sessions, participants were encouraged to communicate their experiential transition from traditional computer screens to the virtual reality domain. This reflective exploration was systematically captured through a questionnaire. Especially, to maintain the integrity and confidentiality of participant responses, survey results underwent a thorough anonymization process through questionnaire forms.
Results
Survey Results
This section presents a comprehensive overview of the survey and its results from participants in the study. Table 1 provides a structured summary of the questions posed to the students. The second column of the table shows the intention behind each question. Additionally, Figures 7, 8 complement the tabulated data, offering visual insights into the patterns and trends discerned from the survey responses. Together, these elements serve as a rich repository of information, unraveling the dynamics of the participants’ engagement with XR technology in the realm of geoscience education.
All questions could be answered in multiple choice, Figures 7, 8 show the outcome of the questionnaire for each of the questions.
In response to Question (A), participants’ previous experience with Virtual Reality (VR) headsets was explored to discern its potential influence on the learning curve in XR training. The results indicated a diverse range of prior experiences, with 60% of participants having used XR before, providing valuable context for their engagement.
Addressing Question (B), opinions on the educational impact of computer programming and simulation in a virtual environment were required. The findings revealed a positive consensus, with a majority agreeing that such experiences foster independent learning through intuitive and interactive simulations.
Question (C) examined into participants’ experiences of motion sickness during VR navigation. The responses unveiled a nuanced landscape, with some reporting discomfort. Further analysis indicated that 15% of participants experienced motion sickness during VR navigation, prompting the need for further investigation to understand causative factors. Note that motion sickness may have been mitigated by applying Mixed Reality (MR) headsets that were not available at the time of this study.
For Question (D), participants’ preference to repeat the VR experience was probed. The majority expressed a willingness to revisit the immersive encounter, reflecting an overall positive response.
Question (E focused on evaluating support for integrating computer programming and simulation in geoscience education. Most participants endorsed this integration, emphasizing its significance for improving teaching and learning quality.
In Question (F), participants were prompted to choose their familiarity with working with subsurface data. The results provided a contextual organization for their experiences, with 76.9% expressing familiarity with 3D seismic data.
Question (G) centered on participants’ satisfaction with seismic visualization in VR. Overall, the visualization of core data and seismic information received positive feedback, with 50% expressing satisfaction with the seismic data visualization improvement.
The subsequent questions (H through J) gave insights into participants’ preferences, confidence levels, and broader views on the use of computer technology in geoscience learning. Generally, participants rated XR as an extremely useful tool for geoscience education, with strong support for the integration of computing programming and simulation.
Based on the responses, it was observed that participants with prior XR experience tended to express higher confidence levels. However, some respondents, regardless of experience, reported motion sickness during VR navigation.
Key findings indicate that the majority (100%) of participants view VR as a valuable tool for enhancing the learning and teaching experience in geosciences. Positive responses, willingness to repeat the experience (100%), and support for integrating computing in education (80%) underscore the potential benefits of VR technology in geoscience education.
Discussion
The integration of VR technology has proven particularly important in the context of teaching and learning activities (Chang et al., 2020; van Bever Donker et al., 2023; Roemmele and Pantazes, 2023). Our study revealed a significant enhancement in students’ comprehension and analysis of seismic data, thereby support the findings of other researches like (Bonali et al., 2021; van Bever Donker et al., 2023; Janiszewski et al., 2020; Jaud et al., 2022; Jitmahantakul and Chenrai, 2019; Kinsland and Borst, 2015; Moysey and Lazar, 2019; Roemmele and Pantazes, 2023; Shin, 2002; Soto et al., 2023; Thurmond et al., 2005). The effectiveness of VR in education is further supported by instances of interactive and immersive exercises integrated into digitized learning spaces.
The potential benefits of the XR platform extend beyond the classroom, providing both students and academics the opportunity for virtual subsurface visualization from a first-person perspective. The positive throughout response from participants during immersive XR sessions, highlights the efficacy of XR visualization. Core data visualization and seismic information earned favourable feedback, emphasizing the value of VR in effectively disseminating geological information.
Moreover, the integration of XR technology not only benefits students but also offers academics the opportunity to engage in virtual subsurface visualization. This approach facilitates the acquisition of diverse geological subsurface knowledge, that might elsewise be impeded by temporal and spatial restrictions. potential benefits may include reduced travel expenses and carbon emissions.
The findings suggest a generally positive perception and acceptance of VR technology among the respondents, with noted satisfaction levels in seismic data visualization. This study indicates that investing in VR technology for geoscience applications has substantial potential benefits in education, exploration, and data analysis within the teaching environment.
For continuous improvement, lessons learned from immersive VR sessions offer valuable insights. Recommendations include expanding the participant pool, incorporating additional VR headsets, and ensuring adequate preparation of seismic data for visualization. This study emphasizes the importance of continuous access to high-resolution photos of core data and the potential enrichment of the collaborative experience through an increased number of VR devices.
Data wise the focus in this study was seismic, accompanied with core data (models of core slabs) and well logs. Here future teaching could include additional data types, such as 3D grids, surfaces, point datasets or also digital outcrops. One of the potential of XR is the ability to display data of different scales in one room.
Other options for future trainings may include training the students in how to present subsurface geoscience data and geoscience stories in XR using their datasets. BaselineZ provides the functionality for creating such stories, which are immersive presentations and allow viewers to interact with all the objects. The students in this study have experienced them. Teaching students how to use the functionality of creating stories would probably require more training time on both using the XR platform, as well as for presenting stories and results in XR, than we have had in this study.
The potential trajectory of this immersive learning initiative also involves engaging a larger group of students, refining the study’s methodology based on lessons learned at Universiti Teknologi Petronas. This iterative process not only ensures continuous enhancement of the platform but also sets the foundation for a more comprehensive and inclusive educational approach. As geoscience education evolves, the strategic integration of VR technology remains decisive in offering enriched educational experiences, facilitating exploration, and advancing data analysis within the academic and teaching environments.
It may be seen by some as a limitation for the teaching approach that we investigated in this study software packages that are commercial. On the other hand, in many cases academic licenses for commercial software are available or a collaborative partnership may be initiated. For geomodeling there may be free and open alternatives, however those may lack compatibility with software used by industry partners. Commercial software has the added advantage of functionality, continuous improvement, and enhanced security, such as SOC2 compliance. For XR in geoscience the authors at the time were not aware of any open-source platform that would allow for replicating the study presented here.
In this study we have not investigated the value of XR in teaching as compared to standard fieldwork outside, in a core shed, or alike or to standard data visualization of geoscience data with the respective desktop applications. However, we have experienced XR as a valuable addition in teaching. This is not for replacing fieldwork nor standard computer applications but for enhancing the students understanding in geoscience. Also, XR makes possible what is elsewise difficult or impossible, such as interacting with real size digital models of full core without feeling their weight, or like inspecting geological models at very large scale. This helps students gain a better understanding for scale, e.g. when comparing a large scale seismic to an original scale core model. We also see a huge potential in XR when traveling to places is difficult or when meeting fellow students or teachers in person is not possible for some reasons. Then XR can take you places and allows for collaboration in an immersive environment with the feeling that “you are really there”.
Conclusion
This study assesses the efficacy of using extended reality for teaching of students at Universiti Teknologi PETRONAS. Throughout the study we used BaselineZ, the spatial computing platform for Geoscience. One focus lies on the students understanding of seismic data also in the context of other geodata. The research examines into the pedagogical viability of this method in geo-education by scrutinizing responses and experiences in virtual reality environment. A prevailing sentiment among the students highlights their keen enthusiasm for the immersive setting, particularly appreciating the virtual approach to teaching and learning geological and seismic concepts. The collective agreement among participants accentuates the pivotal role of virtual reality in elevating the overall learning experience in the field of earth science.
A group of undergraduate students experienced geoscience data visualization, employing Extended Reality (XR). The data, presented through an immersive 3D, holographic view, was made accessible through diverse devices such as mobile devices like phones and tablets as well as VR headsets. This Spatial computing platform facilitated collaborative visualization of multiscale geoscience data within a unified data room.
The students benefit from multi scale research data, here a case study around the EX Field, offshore Malaysia, containing cores, logs, sedimentological descriptions, and seismic data. Beyond its role in enabling joint visualization, the technology played a fundamental role in the generation, presentation, and examination of 3D geoscience stories. The interactive and collaborative nature of the XR platform is particularly supportive for teaching, and it leads to an enriched understanding of intricate geoscientific data.
In consideration of the practical utility of the training, this immersive approach proves to be notably beneficial for geoscience students handling ith complex geological and seismic concepts. The virtual bird’s-eye view teaching methodology, coupled with collaborative visualization through XR technology, offers a distinctive and effective learning experience, especially conducive to comprehending the complexities of multiscale geoscience data.
Looking forward, Universiti Teknologi PETRONAS is contemplating the integration of XR training into its educational framework, highlighting the escalating acknowledgment of the effectiveness of immersive technologies in enhancing the educational landscape. This forward-looking perspective not only demonstrates a commitment to remaining at the vanguard of educational methodologies but also indicates a promising avenue for further exploration and integration of immersive learning experiences within the geoscience curriculum.
Data Availability Statement
The raw data provided for this study is courtesy of Malaysian Petroleum Management (MPM) of PETRONAS and is confidential, bound by the data-sharing agreement between UTP and MPM.
Ethics Statement
Permission to undertake this research was obtained from the authors’ institution, the Center for Subsurface Imaging at Universiti Teknologi Petronas. The study adhered to the relevant research ethics guidelines and regulations to ensure the ethical treatment of participants and the integrity of the research.
Author Contributions
GJ and AL participated in the design of the study, GJ, and KS conducted and prepared the 3D Virtual environments, GJ performed the data collection and interpretation, GJ and KS wrote the manuscript with support of AL. All authors contributed to the article and approved the submitted version.
Funding
The author(s) declare that no financial support was received for the research, authorship, and/or publication of this article.
Conflict of Interest
Author KS was employed by Craytive Technologies.
The remaining author declares that the research was conducted in the absence of any commercial or financial relationships that could be construed as a potential conflict of interest.
Publisher’s Note
All claims expressed in this article are solely those of the authors and do not necessarily represent those of their affiliated organizations, or those of the publisher, the editors and the reviewers. Any product that may be evaluated in this article, or claim that may be made by its manufacturer, is not guaranteed or endorsed by the publisher.
Acknowledgments
The authors extend their appreciation for the invaluable support received from the Centre for Subsurface Imaging, which offered essential facilities for the execution of this research. This study received funding from PETRONAS through the YUTP-FRG Grant titled "Integrated Carbon Storage Potential Assessment in Karst Formations through Immersive Virtual Reality". The research was also supported by software provision by Craytive Technologies, and Baker Hughes. The authors are also very grateful to Stephen Stein for his insightful review and constructive comments, which significantly contributed to the improvement of this paper. Likewise, we thank the reviewers Emeritus Professor Gary L. Kinsland and Professor Jennifer M. McKinley for their careful reviewing and commenting, which helped to further improve the manuscript.
References
Apple (2024). Apple Vision Pro. Available at: https://www.apple.com/apple-vision-pro/.
Baker Hughes (2022). JewelSuite Subsurface Modeling. Available at: https://www.bakerhughes.com/oilfield-services-and-equipment-digital/well-planning/jewelsuite-subsurface-modeling.
Barco (2023). Barco. Available at: https://www.barco.com/.
Bonali, F. L., Russo, E., Vitello, F., Antoniou, V., Marchese, F., Fallati, L., et al. (2021). How Academics and the Public Experienced Immersive Virtual Reality for Geo-Education. Geosciences 12 (1), 9. doi:10.3390/geosciences12010009
Chang, S. C., Hsu, T. C., Kuo, W. C., and Jong, M. S. Y. (2020). Effects of Applying a VR-based Two-Tier Test Strategy to Promote Elementary Students’ Learning Performance in a Geology Class. Br. J. Educ. Technol. 51 (1), 148–165. doi:10.1111/bjet.12790
Craytive Technologies BV. Solutions (2023). BaselineZ. Available at: https://baselinez.com/.
Ellis (2019). Paleoscan. Available at: https://www.eliis-geo.com/paleoscan-overview-a.html.
Epting, M. (1980). Sedimentology of Miocene Carbonate Buildups, Central Luconia, Offshore Sarawak. Bull. Of Geol. Soc. Malays. 12, 17–30. doi:10.7186/BGSM12198002
Gartner, G. L. B., Schlager, W., and Adams, E. W. (2004). Seismic Expression of the Boundaries of a Miocene Carbonate Platform. Malaysia: Sarawak.
Igloo Vision (2023). Igloo Vision | the Shared Immersive Space Company. Available at: https://www.igloovision.com/.
Janiszewski, M., Uotinen, L., Merkel, J., Leveinen, J., and Rinne, M. (2020). “Virtual Reality Learning Environments for Rock Engineering, Geology, and Mining Education,” in ARMA American Rock Mechanics Association (ARMA) (US Rock Mechanics/Geomechanics Symposium (pp. ARMA-2020): ARMA). Available at: https://onepetro.org/ARMAUSRMS/proceedings-abstract/ARMA20/All-ARMA20/447531.
Jaud, M., Geoffroy, L., Chauvet, F., Durand, E., and Civet, F. (2022). Potential of a Virtual Reality Environment Based on Very-High-Resolution Satellite Imagery for Structural Geology Measurements of Lava Flows. J. Struct. Geol. 158, 104569. doi:10.1016/j.jsg.2022.104569
Jiménez Soto, G., Abdul Latiff, A. H., Ben Habel, W., Bing Bing, S., and Poppelreiter, M. (2022). Integrated Carbonate Rock Type Prediction Using Self-Organizing Maps in E11 Field, Central Luconia Province, Malaysia. Appl. Sci. 12 (15), 7688. doi:10.3390/APP12157688
Jitmahantakul, S., and Chenrai, P. (2019). Applying Virtual Reality Technology to Geoscience Classrooms, Review of International Geographical Education Online. RIGEO 9, 577–590. doi:10.33403/rigeo.592771
Kinsland, G. L., and Borst, C. W. (2015). Visualization and Interpretation of Geologic Data in 3D Virtual Reality. Interpretation 3 (3), SX13–SX20. doi:10.1190/int-2014-0252.1
Lee, Y., and Yoo, B. (2021). XR Collaboration Beyond Virtual Reality: Work in the Real World. J. Comput. Des. Eng. 8 (2), 756–772. doi:10.1093/jcde/qwab012
Meta (2023). Metaquest. Available at: http://meta.com/quest/quest-3.
Microsoft (2019). Hololens. Available at: https://www.microsoft.com/en-us/hololens.
Milgram, P., and Kishino, F. (1994). A Taxonomy of Mixed Reality Visual Displays. IEICE Transactions on Information and Systems. E77-D, 1321–1329.
Moysey, S. M. J., and Lazar, K. B. (2019). “Using Virtual Reality as A Tool for Field-Based Learning in the Earth Sciences,” in Interdisciplinary Perspectives on Virtual Place-Based Learning, 99–126.
Onajite, E. (2013) “Seismic Data Analysis Techniques in Hydrocarbon Exploration,” in Seismic Data Analysis Techniques in Hydrocarbon Exploration, 1–237. doi:10.1016/C2013-0-09969-0
Rankey, E. C., Schlaich, M., Mokhtar, S., Ghon, G., Ali, S. H., and Poppelreiter, M. (2019). Seismic Architecture of a Miocene Isolated Carbonate Platform and Associated Off-Platform Strata (Central Luconia Province, Offshore Malaysia). Mar. And Petroleum Geol. 102, 477–495. doi:10.1016/j.marpetgeo.2019.01.009
Roemmele, C., and Pantazes, T. (2023). “Creating and Utilizing Virtual Reality and Video Demonstrations to Support Student Learning and Understanding of Geoscience,” in Conference Proceedings. New Perspectives in Science Education 2023.
Rossi, P., Mancini, F., Dubbini, M., Mazzone, F., and Capra, A. (2017). Combining Nadir and Oblique UAV Imagery to Reconstruct Quarry Topography: Methodology and Feasibility Analysis. Eur. J. Remote Sens. 50 (1), 211–221. doi:10.1080/22797254.2017.1313097
Schlumberger (2019). Petrel. Available at: https://www.software.slb.com/products/petrel.
Schmitz, B., Holst, C., Medic, T., Lichti, D. D., and Kuhlmann, H. (2019). How to Efficiently Determine the Range Precision of 3D Terrestrial Laser Scanners. Sensors 19 (6), 1466. doi:10.3390/s19061466
Shin, Y. S. (2002). Virtual Reality Simulations in Web-Based Science Education. Comput. Appl. Eng. Educ. 10 (1), 18–25. doi:10.1002/cae.10014
Soto, G. J., Schulze, K., and Latiff, A. H. (2023). Effectiveness and Usability of Subsurface Geodata Visualization for Training and Storytelling Using Virtual Reality: Immersing into A Dataset from the EX-Carbonate Field in Central Luconia Province (Malaysia). EGU23. doi:10.5194/EGUSPHERE-EGU23-719
Thurmond, J. B., Drzewiecki, P. A., and Xu, X. (2005). Building Simple Multiscale Visualizations of Outcrop Geology Using Virtual Reality Modeling Language (VRML). Comput. & Geosciences 31 (7), 913–919. doi:10.1016/j.cageo.2005.03.007
van Bever Donker, J., Marshal, D., Huber, M., Maart, R., Mayekiso, L., Solomon, H., et al. (2023). The Effectiveness of Using Virtual Reality Materials in Preparing Students for Geological Fieldwork (No. EGU23-13788). Copernicus Meetings.
Keywords: virtual reality, geoscience education, seismic data, petroleum geoscience, 3D virtual environments
Citation: Jiménez G, Latiff AH and Schulze K (2024) Subsurface Geosciences Learning in Virtual Reality: A Case Study in Central Luconia Province, Malaysia. Earth Sci. Syst. Soc. 4:10118. doi: 10.3389/esss.2024.10118
Received: 27 February 2024; Accepted: 26 August 2024;
Published: 07 October 2024.
Edited by:
Jennifer M. McKinley, Queen’s University Belfast, United KingdomReviewed by:
Gary Kinsland, University of Louisiana at Lafayette, United StatesJennifer M. McKinley, Queen’s University Belfast, United Kingdom
Copyright © 2024 Jiménez, Latiff and Schulze. This is an open-access article distributed under the terms of the Creative Commons Attribution License (CC BY). The use, distribution or reproduction in other forums is permitted, provided the original author(s) and the copyright owner(s) are credited and that the original publication in this journal is cited, in accordance with accepted academic practice. No use, distribution or reproduction is permitted which does not comply with these terms.
*Correspondence: Grisel Jiménez, amltZW5lenNvdG9ncmlzZWxAZ21haWwuY29t
†Present address: Grisel Jiménez, Petronas Carigali, Kuala Lumpur, Malaysia